Recent advances in transesterification for sustainable biodiesel production, challenges, and prospects: a comprehensive review
- Review Article
- Open access
- Published: 23 January 2024
- Volume 31 , pages 12722–12747, ( 2024 )

Cite this article
You have full access to this open access article
- Sabah Mohamed Farouk ORCID: orcid.org/0009-0000-8907-5954 1 ,
- Aghareed M. Tayeb 2 ,
- Shereen M. S. Abdel-Hamid 1 &
- Randa M. Osman 3
5562 Accesses
11 Citations
Explore all metrics
Biodiesel, a renewable and sustainable alternative to fossil fuels, has garnered significant attention as a potential solution to the growing energy crisis and environmental concerns. The review commences with a thorough examination of feedstock selection and preparation, emphasizing the critical role of feedstock quality in ensuring optimal biodiesel production efficiency and quality. Next, it delves into the advancements in biodiesel applications, highlighting its versatility and potential to reduce greenhouse gas emissions and dependence on fossil fuels. The heart of the review focuses on transesterification, the key process in biodiesel production. It provides an in-depth analysis of various catalysts, including homogeneous, heterogeneous, enzyme-based, and nanomaterial catalysts, exploring their distinct characteristics and behavior during transesterification. The review also sheds light on the transesterification reaction mechanism and kinetics, emphasizing the importance of kinetic modeling in process optimization. Recent developments in biodiesel production, including feedstock selection, process optimization, and sustainability, are discussed, along with the challenges related to engine performance, emissions, and compatibility that hinder wider biodiesel adoption. The review concludes by emphasizing the need for ongoing research, development, and collaboration among academia, industry, and policymakers to address the challenges and pursue further research in biodiesel production. It outlines specific recommendations for future research, paving the way for the widespread adoption of biodiesel as a renewable energy source and fostering a cleaner and more sustainable future.
Similar content being viewed by others
Biodiesel: Features, Potential Hurdles, and Future Direction
Assessment of Catalytic Biodiesel Production: A Mini-Review
Biodiesel: Use of Green Feedstocks and Catalysts
Explore related subjects.
- Environmental Chemistry
Avoid common mistakes on your manuscript.
Introduction
Fossil fuels are responsible for most of the world’s energy needs, endangering the environment. The consumption of fossil fuel products like coal and petroleum has increased because of ongoing globalization and industrialization (Touqeer et al. 2020 ). Around 580 TJ of energy is needed annually for the entire planet, and a staggering 80% of that energy is supplied by burning traditional fossil fuels. Greater energy use is required by expanding the population and improving accessibility, which increases the depletion of fossil fuel reserves (Martchamadol and Kumar 2012 ). Finding an alternate energy source, like biodiesel, is essential for improving energy security for economic development (Oh et al. 2002 ; Um and Kim 2009 ). Biodiesel is a renewable, sustainable, and biodegradable fuel with low greenhouse gas emissions (Sharma and Singh 2009 ; Lee et al. 2011 ). Oils from plants and animals, as well as other lipids such as triacylglycerides (TAGs), can be converted into biodiesel (Hoekman and Robbins 2012 ). The process of making biodiesel, called transesterification or alcoholysis, typically requires the use of catalysts such as acids, bases, and enzymes (Ong et al. 2014 ). Catalysts can exist in either a homogeneous or heterogeneous phase. A homogeneous catalyst undergoes alcoholysis in the same phase, often liquid, as the reactants. In contrast, a heterogeneous catalyst is in a different phase, often one that is not liquid, from the reactants (Ruhul et al. 2015 ). Nanomaterials have become increasingly important in enhancing the production of biodiesel. The weight of the catalyst, the reaction temperature, and the oil-to-alcohol ratio are all reduced when a nanocatalyst is used (Dhawane et al. 2018 ; Ghosh and Halder 2022 ). It has already been proven that nanocatalysts play a key role in increasing response velocity. Additionally, it significantly lowers the reactants’ activation energy. This study addresses the need to keep up with the rapidly changing field of biodiesel production. By conducting thorough surveys and reviews, researchers can stay informed about the latest advancements and challenges. This approach helps focus research efforts by identifying promising methodologies and comparing their performance. The production of biodiesel encounters several challenges, including costly feedstocks, environmental concerns, and the quest for improved efficiency. Through a meticulous review of relevant literature, researchers can identify these challenges and propose potential solutions. This review serves as a valuable resource, synthesizing information from different sources to provide a comprehensive understanding of the current state of biodiesel production technology. Policymakers and industry leaders rely on up-to-date insights to make informed decisions that shape the future of the biodiesel industry. Concise surveys and reviews effectively communicate the latest information, enabling informed decision-making. Additionally, this review promotes collaboration and knowledge sharing within the biodiesel research community, facilitating the development of new and better technologies. It also educates the public about biodiesel production and its potential benefits, potentially increasing support and encouraging the adoption of biodiesel as a sustainable alternative to petroleum-based fuels. Literary, and in detail, this paper offers a comprehensive review of biodiesel production, encompassing key topics such as feedstock selection, the transesterification process, the recovery and reusability of nanoparticles, the benefits and challenges of biodiesel use in engines, and the significance of techno-economic analysis. It begins with various feedstocks, including used oils, animal fats, algae, and edible and inedible vegetable oils, which are chosen for their potential to produce biodiesel. The review then highlights biodiesel as a promising alternative to fossil fuels, exploring its environmental benefits, renewability, and potential to reduce dependence on fossil fuels. The transesterification process, a crucial step in biodiesel production, is discussed in detail, covering reaction mechanisms, catalysts, influencing factors, and challenges associated with optimization. Additionally, various methods and strategies, including solid supports, magnetic separation, centrifugation, and surface modification, have been explored for the recovery and reusability of nanoparticles as catalysts in biodiesel production. These approaches offer promising prospects for enhancing the efficiency and sustainability of biodiesel synthesis while reducing costs and environmental impacts. The benefits of using biodiesel in engines, including improved lubricity, reduced emissions, and potential performance enhancements, are discussed. Lastly, this review emphasizes the importance of techno-economic analysis in assessing the financial viability and sustainability of biodiesel production, considering factors such as capital investment, operating costs, feedstock prices, and governmental policies. Overall, this comprehensive review serves as a valuable resource for researchers, engineers, and policymakers in the biodiesel industry.
Feedstocks for biodiesel production
Biodiesel can be synthesized from various raw materials, including vegetable oils, animal fats, algae, and waste cooking oil. Each feedstock has its own advantages and challenges regarding availability, cost, sustainability, and energy content. The purpose of using different raw materials is to diversify the sources of renewable energy and reduce dependence on fossil fuels. At least 80% of the current costs of producing biodiesel come from the feedstock (Sayed and El-Gharbawy 2016 ). Given the global food crisis, almost 95% of biodiesel produced worldwide is manufactured from edible oils, which are viewed as unnecessary (Azizian and Kramer 2005 ). As a result, making biodiesel from inexpensive, non-edible oil is the current trend (Balat 2011 ). A few feedstocks, including palm, jatropha, microalgae, coconut tallow, and used cooking oil, stand out for their high productivity in the manufacturing of biodiesel (Gui et al. 2008 ). Figure 1 represents some different feedstocks for biodiesel production.
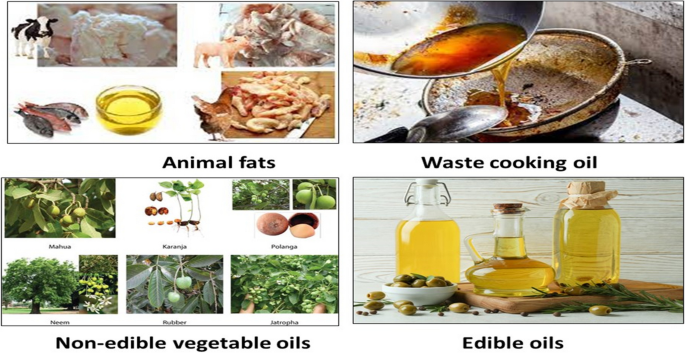
The choice of raw materials has a significant impact on the production of biodiesel in terms of yield, quality, cost, and environmental sustainability. The fatty acid profile of the raw material plays a crucial role in determining the properties and performance of biodiesel. Oils with high levels of saturated fatty acids produce biodiesel with better cold flow properties, while oils with high levels of unsaturated fatty acids produce biodiesel with better oxidative stability (Suzihaque et al. 2022 ). FFA content significantly impacts the reaction rate and biodiesel yield. Higher FFA content requires pre-treatment steps, such as esterification, to reduce acidity and prevent soap formation, which can hinder the reaction and reduce biodiesel purity (Javidialesaadi and Raeissi 2013 ). The amount of water in the raw material can also affect the transesterification reaction. Excess water can hydrolyze the fatty acid methyl esters produced during the reaction, reducing biodiesel yield. Therefore, feedstocks with low water content are preferred (Atadashi et al. 2012 ). The overall quality of the raw material, including its purity and the presence of impurities, can influence biodiesel production. Impurities like phospholipids, gums, and waxes can interfere with the reaction and complicate downstream processing (Manzanera et al. 2008 ). The environmental impact of raw material sourcing should be considered. Using waste cooking oil or non-edible oils reduces the competition for land with food production and minimizes the environmental footprint of biodiesel production. The price of the raw material is a major factor affecting the economics of biodiesel production. Utilizing inexpensive feedstocks, such as waste cooking oil or locally produced oils, can significantly reduce production costs. The choice of raw material may necessitate adjustments in processing conditions, such as reaction temperature, catalyst concentration, and reaction time, to optimize biodiesel yield and quality. Byproducts generated during biodiesel production, such as glycerol, can be utilized for various applications, adding value to the overall process and reducing waste. The sourcing of raw materials can have social and economic impacts on local communities. Promoting the use of locally available or waste-based feedstocks can stimulate agricultural economies and create employment opportunities (Srivastava et al. 2018 ; Suzihaque et al. 2022 ). In conclusion, the selection of raw materials for biodiesel production requires a careful assessment of their fatty acid profile, FFA content, water content, overall quality, environmental sustainability, cost-effectiveness, and potential for byproduct utilization. By considering these factors, biodiesel production can be optimized for yield, quality, cost, and environmental sustainability. Devaraj et al. ( 2020 ) created biodiesel from used frying oil. At 75 °C, 1 wt% catalyst concentration, 1:6 oil-to-methanol molar ratio, 350 rpm, and 90 min, 97% of the possible biodiesel output was produced. In the pilot plant, biodiesel was also produced under these process conditions, with a 97% yield. Hamed et al. ( 2021 ) converted Afia waste cooking oil (AWCO) into biodiesel fuel. At the ideal working conditions of 60 °C reaction temperature, 3 h reaction time, and 0.4 catalyst concentration, the maximum conversion and yield of biodiesel are 97.54 and 94.935%, respectively. Roy et al. ( 2021 ) reported on the conversion of used frying oil into biodiesel to manage liquid waste. The optimum conditions for the transesterification of WFO were 1:16 oil to methanol by weight, 3 wt% catalyst, 65 °C reaction temperature, and 35 min reaction time. FAME conversion (99.5%) and 96% yield are achieved at this optimal reaction setting. Karmakar and Halder ( 2021 ) researched the transesterification reaction used in supercritical settings to produce biodiesel fuel from fish waste oil. According to calculations, the experimental yield of biodiesel generation under ideal conditions was 94.6%. Al Hatrooshi et al. ( 2020 ) created the fatty acid methyl ester from waste shark liver oil (WSLO). At a methanol-to-WSLO ratio of 10.3 M, a reaction duration of 6.5 h, a temperature of 60 °C, and a catalyst concentration of 5.9 wt%, acid-catalyzed WSLO transesterification achieved 99% FAME conversion. A biodiesel yield of 99.73% was achieved at 60 °C for 1.5 h using a 1.2% catalyst and a 6:1 methanol:oil ratio. In a batch-stirred reactor, Nisa et al. ( 2020 ) conducted an experiment to produce biodiesel from the microalgae Spirulina sp. using 1 wt% (w/w) of palm oil as a co-solvent for methanol and potassium hydroxide. At 60 °C, with a methanol-to-palm oil molar ratio of 10:1 and a palm oil-to-microalgae weight ratio of 5:1, the best biodiesel production of 85.28% was generated. Olubunmi et al. ( 2020 ) converted the bio-oil from dairy scum waste into biodiesel. Using a 9:1 methanol-to-oil molar ratio, 40 min for the reaction, 65 °C for the reaction temperature, and 300 rpm for the mixing speed, the maximum biodiesel yield of 94.8% was achieved. In India, Jain and Sharma ( 2010 ) utilized Jatropha oil for the manufacture of biodiesel under the ideal conditions of a 3:7 (v/v) methanol-to-oil ratio and a 1% (w/w) catalyst concentration for mixing at 400 rpm with H 2 SO 4 and NaOH. The transesterification of pretreated JCO yielded the highest yield of 90.1%. Abdulrahman ( 2017 ) made a biodiesel fuel from used cooking oil and chicken fat. The highest conversion to ester was attained at a methanol-to-oil ratio of roughly 7:1 at 60 °C and an 83% yield, also Al-Mawaali et al. ( 2023 ) utilized discarded animal fats and used cooking oil as a cheap source of feedstock to produce biodiesel. With NaOH acting as a catalyst, the discarded frying oil produced the highest yield of synthesized biodiesel (80.6%), followed by a mixture of waste cooking oil and animal fats (79.3%). Finally, using multiple raw materials as feedstock for biodiesel synthesis helps to diversify energy sources, reduce dependency on fossil fuels, and promote sustainability. Every feedstock source has benefits and drawbacks, and the choice of feedstock is influenced by factors like cost, sustainability, availability, and the needs of the biodiesel production process. Continued R&D efforts are aimed at enhancing the efficiency, cost-effectiveness, and sustainability of biodiesel production from diverse feedstocks.
Feedstock preparation
Cleaning and drying: The feedstock is cleaned to get rid of pollutants, water, and debris. It could entail processes like sedimentation or filtration. The feedstock is dried to eliminate any last traces of moisture after cleaning (Sait et al. 2022 ).
Pretreatment: Pretreatment of feedstocks may be necessary in some situations to enhance their quality and appropriateness for the manufacture of biodiesel. Degumming, acid esterification, or neutralization are some examples of pretreatment techniques used to get rid of pollutants, gums, and free fatty acids (Singh et al. 2011 ).
The most popular method of treatment for reducing FFAs is acid esterification. One efficient method for lowering FFAs is glycerolysis, also known as glycerol esterification (Elgharbawy et al. 2021 ). Hayyan et al. ( 2011 ) treated the high FFA percentage in palm oil using sulfuric acid. They used H 2 SO 4 by 0.75 wt%, a methanol-to-oil molar ratio of 8:1, 60 min of reaction time, and 60 °C to successfully reduce the FFA content from 23 wt% to less than 2 wt%. Kara et al. ( 2018 ) examined how various methanol-to-oil molar ratios affected the final FFA% throughout the esterification reaction while maintaining constant values for other parameters. Within 160 min of the reaction, the ideal conditions were reached at a molar ratio of 15:1. The highest conversion of 92.6% was achieved, while the FFA content decreased from 21 to 1.5%. Sadaf et al. ( 2018 ) investigated how three acids HCl, H 2 SO 4 , and H 3 PO 4 affected used cooking oil containing 2.75 wt% FFAs. At 60 °C and a 2.5:1 methanol-to-oil molar ratio, the FFA dropped to 0.33 wt%, indicating that H 2 SO 4 was the most effective catalyst. Sousa et al. ( 2010 ) treated castor oil with a non-catalytic glycerolysis procedure with a glycerol-to-oil mass ratio of 1:1 and an FFA of 2.5 wt% for 2 h at 120 °C. They were able to reduce the FFAs from 2.5 to 0.2 wt%.
Triglyceride content in different feedstocks
Triglycerides are the primary component of biodiesel and are present in various amounts in the feedstocks used in the process. Mono- and diglycerides, free fatty acids, phosphatides, sterols, fatty alcohols, fat-soluble vitamins, and other compounds are among the minor constituents. The amount of triglycerides in the feedstock has a significant impact on the output and caliber of biodiesel produced. Most dietary fats and oils are mostly composed of triglycerides.
Vegetable oils
Various vegetable oils, including canola, sunflower, soybean, and palm oils, are frequently utilized as feedstocks in the manufacturing of biodiesel. High concentrations of triglycerides, which are made up of three fatty acid chains joined to a glycerol backbone, are found naturally in these oils. Vegetable oils, therefore, have a high triglyceride concentration, usually more than 90% by weight. Vegetable oils are ideal feedstocks for the synthesis of biodiesel because of their high triglyceride content. Because refined vegetable oils convert pure triglycerides (TG) to FAME at a high rate and quickly, they are the ideal feedstock for producing biodiesel.
Animal fats
Fish oil, tallow, lard, and chicken fat are examples of animal fats that can be utilized as feedstocks to produce biodiesel. Comparing animal fats to vegetable oils, the former usually have a lower triglyceride concentration, usually between 70 and 85% by weight. The species of the animal, its nutrition, and the rendering techniques all affect the variation in triglyceride content. Animal fats can still be effectively turned into biodiesel even when their triglyceride level is slightly lower.
Waste oils and greases
The manufacturing of biodiesel can use recycled waste cooking oils and greases from food processing industries, restaurants, and other sources as feedstocks. Depending on their quality and place of origin, waste oils and greases might include varying amounts of triglyceride. The quality and conversion efficiency of biodiesel can be impacted by the presence of contaminants or elevated quantities of free fatty acids in these feedstocks.
Algae and microorganisms
The capacity of algae and specific microorganisms to accumulate large concentrations of lipids (fatty acids and triglycerides) makes them viable feedstocks for the generation of biodiesel. Depending on the species and growing environment, triglycerides can make up a large portion of algal oils, anywhere from 20 to 60% by weight. Table 1 represents the triglyceride content of different feedstocks.
Biodiesel as a promising alternative source of biofuel
Among the emerging alternatives, biodiesel has garnered significant attention as a promising biofuel with the potential to reduce reliance on petroleum-based fuels and mitigate environmental impacts. The non-toxic, biodegradable fuel known as biodiesel is made from leftover cooking oil, animal fats, or vegetable oil, and presents several advantages over conventional diesel. Firstly, its renewable nature alleviates concerns over depleting fossil fuel resources. Unlike petroleum diesel, which is extracted from finite underground reservoirs, biodiesel can be produced from continuously replenished sources, ensuring long-term sustainability. Secondly, biodiesel boasts superior environmental credentials compared to diesel. Its burning emits significantly lower degrees of particulate matter, nitrogen oxides, sulfur oxides, and carbon monoxide, all of which contribute to air pollution and its detrimental effects on human health and the environment. Additionally, biodiesel’s lower greenhouse gas emissions make it a promising alternative for reducing the transportation sector’s contribution to climate change (Demirbas 2009 ). Moreover, biodiesel offers economic benefits, particularly for regions with abundant feedstock sources. Local production of biodiesel can stimulate agricultural economies, reduce reliance on imported petroleum, and create employment opportunities. Additionally, biodiesel’s compatibility with existing diesel engines eliminates the need for expensive infrastructure overhauls, facilitating a smoother transition toward a more sustainable fuel source (Fukuda et al. 2001 ). Despite its promise, biodiesel faces certain challenges that need to be addressed for its widespread adoption. One challenge lies in its economic viability. The production cost of biodiesel is currently higher than that of petroleum diesel, primarily due to feedstock costs and processing expenses. However, advancements in production technologies and economies of scale are expected to reduce biodiesel costs over time.
Another challenge involves the availability of suitable feedstocks. While various plant oils, such as soybean, palm, and jatropha, can be used for biodiesel production, concerns have arisen regarding land-use competition for the food industry and the potential environmental effects of broad-scope palm oil plantations. Sustainable feedstock sourcing strategies, such as utilizing waste cooking oil and cultivating oil crops on marginal lands, are crucial to addressing these concerns (Knothe 2010 ; Singh and Singh 2010 ). In conclusion, biodiesel presents a compelling alternative to conventional diesel, offering a combination of sustainability, environmental benefits, and economic potential. Addressing the current challenges related to feedstock availability and production costs will pave the way for biodiesel’s wider adoption and its significant contribution to a more sustainable energy future.
Innovations in biodiesel applications
Biodiesel, a renewable and cleaner-burning alternative to conventional diesel fuel, has gained significant attention and widespread applications in recent years. Its versatile nature allows for various uses across different sectors. In transportation, biodiesel can be blended with petroleum diesel to power vehicles, reducing emissions of greenhouse gases and air pollutants. Moreover, biodiesel finds applications in industrial settings, where it serves as a substitute for petroleum-based fuels in machinery and equipment. The growing interest in sustainable energy solutions has spurred research and development efforts, leading to advancements in biodiesel production techniques, feedstock selection, and engine compatibility. Recent studies (Mobin et al. 2022 ; Tripathi et al. 2023 ) have explored the potential of advanced feedstocks, such as algae and waste oils, to enhance biodiesel production efficiency and reduce environmental impacts. These advancements in biodiesel applications and technology are crucial steps in achieving a more sustainable and greener energy future. Figure 2 shows some of the applications of biodiesel.
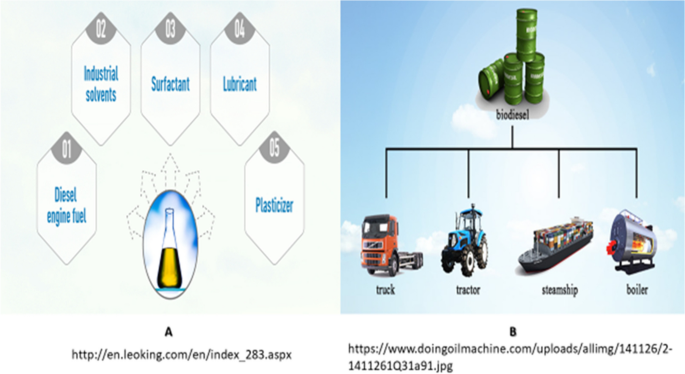
Biodiesel applications
Transesterification in biodiesel production
Alcohol and lipids react chemically to produce fatty acid alkyl esters, a process known as transesterification. Triglycerides and alcohol are transesterified to produce FAAE and glycerol. Triglycerides and alcohols combine to form diglycerides in the first stage, which are then converted to monoglycerides and glycerol, each of which yields an alkyl ester (Thangaraj et al. 2019 ). Among the factors influencing biodiesel yield in transesterification are time, temperature, type and concentration of the catalyst, kind of feedstock oil, and alcohol-to-oil ratio. It is possible to reverse the transesterification process. To shift the reaction’s equilibrium in favor of the product’s production, an excess of alcohol is therefore necessary. Alcohols with short chains, long chains, and cyclic chains are all used in this process. The availability, polarity, better reactivity, and inexpensive price of methanol and ethanol, however, make them popular choices (Avhad and Marchetti 2015 ), Fig. 3 illustrates the characteristics and features of the transesterification process used to make biodiesel, and Fig. 4 shows a schematic representation of the biodiesel synthesis path through the transesterification process.
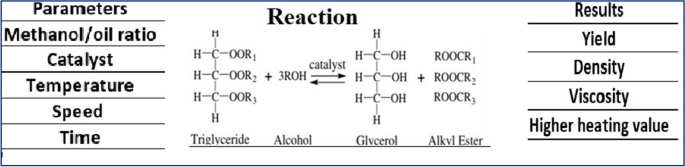
The characteristics and features of the transesterification process used to make biodiesel
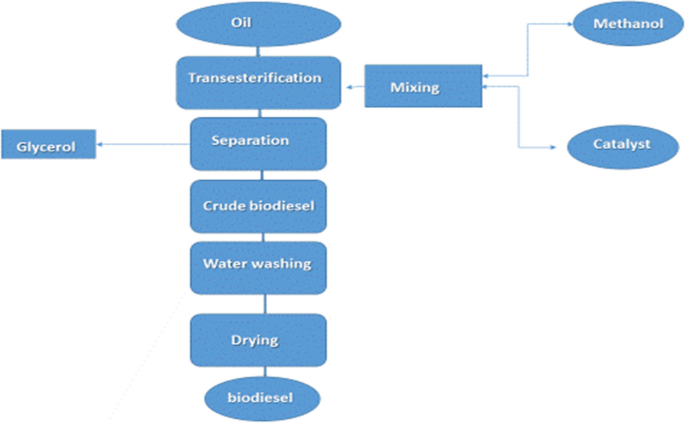
Schematic representation of the biodiesel synthesis path through the transesterification process
Triglycerides are changed into diglycerides, monoglycerides, and finally glycerol by a sequence of chemical events called transesterification, which is the process used to produce biodiesel. Alkali catalysts are usually used in a single phase of this process. However, a two-step procedure can be required if the feedstock has large concentrations of water or free fatty acids (FFAs). Fatty acid esters, or acid-catalyzed alcoholysis, are the initial step that turns FFAs into fatty acid esters. The process of transesterification, which turns the fatty acid esters into biodiesel, comes next. Figure 5 displays the schematic diagram for the manufacture of biodiesel in both one and two steps.
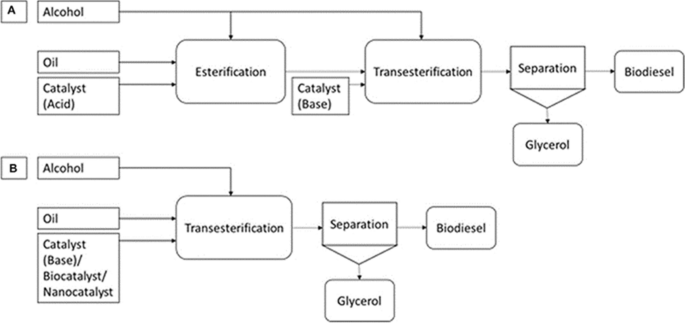
Biodiesel manufacturing can be done in two steps ( A ) or one step ( B ) (Fattah et al. 2014 ; Mofijur et al. 2014 )
Chemical or biological catalysts can be used in transesterification reactions that are catalyzed. The classification is shown in Fig. 6 . There are both homogeneous and heterogeneous chemical catalysts. Base or acid catalysts are included in the homogeneous catalyst. The heterogeneous catalyst is made up of nano-, biomass waste-based, base, and acid–base functionalities (Thangaraj et al. 2019 ). The choice of any catalyst is influenced by the following factors: oil quality, FFA content, operating conditions, necessary catalyst activity, cost, and availability (Tacias-Pascacio et al. 2019 ). The advantages and disadvantages of different catalysts are presented in Table 2 .
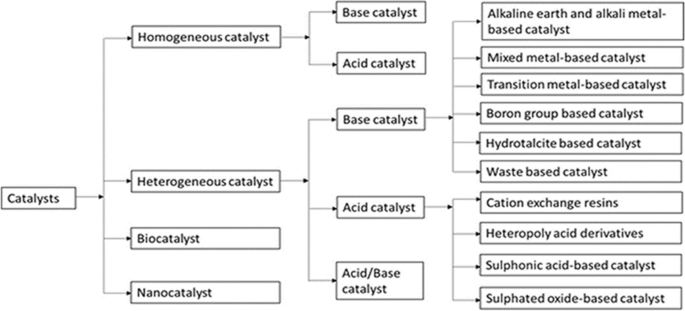
Different catalysts used in biodiesel production (Rizwanul Fattah et al. 2020 )
- Homogeneous catalysis
Homogeneous catalysts are the ones that are most frequently used in the production of biodiesel because they are simple to use and require less time to complete a reaction. When dissolving a homogeneous catalyst, a solvent that is in the same phase as all the reactants is usually utilized (Rizwanul Fattah et al. 2020 ). Bhuana et al. ( 2020 ) used leftover beef tallow and methanol as solvents, in a KOH catalyst to create biodiesel. Ethanol effectively functioned as a co-solvent, lowering reaction time by 61.11% and functioning as a low-polarity active ester exchange agent, which prevented soap formation and increased yield by 3.08%. Karmee et al. ( 2015 ) looked at the transesterification of algal oil using methanol as the solvent. Under identical reactional conditions, HCl outperformed the homogeneous H 2 SO 4 catalyst. Rice bran oil was esterified by Arora et al. ( 2015 ) utilizing sulfuric acid as a uniform catalyst. Studies have been done on how the oil-to-methanol molar ratio (1:5 to 1:30), catalyst concentration (0.15 to 1.0 wt%), and reaction temperature (45 to 60 °C) affect the conversion of FFA. Siddiqua ( 2015 ) transesterified palm oil to create biodiesel. Further observation shows that a mixture of methanol and NaOH at a reaction temperature of 55 °C produced the highest production. Abdulsalam ( 2023 ) assessed the process of turning thevetia peruviana seed oil into biodiesel using two different catalysts (NaOH and KOH). Separate NaOH and KOH pellets were dissolved in methanol to create different catalysts with different amounts of sodium and potassium methoxide, respectively. The NaOH catalyst’s conversion yield was 81.2%, 80.4%, and 89%, whereas the KOH catalyst’s conversion yield was 96.8%, 88.4%, and 84.0. Through the calcination of leftover pineapple leaves, de Barros et al. ( 2020 ) created a unique catalyst. After a 30-min reaction period at 60 °C, 4 wt% of catalyst, a molar ratio of 1:40 for oil to methanol, and an oil-to-biodiesel conversion of above 98%, a high catalytic activity was detected. This activity is likely connected to the 85-wt% presence of alkali/alkali metals (K, Ca, and Mg). Kasirajan ( 2021 ) used two-step procedures to produce biodiesel from Chrysophyllum albidum seed, a non-edible source. Transesterification is performed on oil from Chrysophyllum albidum seeds after esterification employing a homogeneous catalyst of H 2 SO 4 . The maximum oil-to-biodiesel conversion was 99.2 wt% when the ideal situations were achieved, which included a 1:9 oil-to-methanol molar ratio, 1 wt% KOH, 500 rpm, and 40 min at 65 °C. Belkhanchi et al. ( 2021 ) showed that transesterifying used frying oils (UFO) at 18 °C for 60 min of reaction in the presence of methanol using MeOH/UFO 6:1 mol proportion and 1 wt% of KOH yields the best conversion of UFO. Jain et al. ( 2023 ) gave a description of the homogeneous base catalyst-based single-step transesterification process for producing biodiesel from waste cooking oil that contains high levels of free fatty acids and algal oil. According to the findings, a biodiesel yield of 92% may be achieved under optimal conditions, which include a 1.5% catalyst (w/w), a methanol to oil ratio of 21:1, a time of 110 min, and a temperature of 50 °C. Saeed et al. ( 2021 ) investigated S. elongata algal for biodiesel creation. To evaluate transesterification to FAME, zeolitic catalysts, KOH, and HCl were used. KOH produced the maximum biodiesel yield (99.9%), which was obtained under the ideal reaction conditions of a 1.0% catalyst, 60 °C, 4 h, and a 1:4 volume ratio between oil and methanol. Table 3 highlights some of the recently published research on the use of several homogeneous catalyst types for biodiesel synthesis, various feedstock sources, experimental setups, and biodiesel yields.
- Heterogeneous catalysis
Heterogeneous catalysts go through different phases or states than reactants. According to Melero et al. ( 2009 ), these are the catalysts that often generate active sites when reacting with their reactants. Greater oil/alcohol ratios and greater temperatures than in homogeneous catalysis are the primary disadvantages of this catalysis. The catalyst’s improved reusability and ease of separation and purification are other advantages. Mohamed et al. ( 2020 ) prepared by quickly pyrolyzing rice straw, a heterogeneous catalyst (RS-SO 3 H) was created. The yield of biodiesel was 90.37%. in ideal conditions: 20:1 methanol: oil molar ratio with a 10% catalyst at 70 °C for 6 h. Choksi et al. ( 2021 ) created a solid acid catalyst using the sulfonation carbonization process from a palm fruit bunch. After that, the catalyst was put through esterification and transesterification processes to produce biodiesel. Utilizing a 4% catalyst, a 21:1 methanol-to-oil molar ratio, and a 60 °C temperature, an optimal yield of 88.5 wt% methyl ester was obtained in 180 min. Aghel et al. ( 2019 ) wanted to improve a pilot-scale microreactor that used kettle limescale to turn used cooking oil (WCO) into biodiesel. The produced biodiesel had a maximum conversion of 93.41% at 61.7 °C, a catalyst concentration of 8.87 wt %, a methanol-to-oil 1.7:3 volumetric ratio, and 15 min. Bhatia et al. ( 2020 ) developed a heterogeneous catalyst to initiate the transesterification of used cooking oil by pyrolyzing waste cork. The greatest conversion (98%) for the heterogeneous catalyst produced at 600 °C occurred at alcohol:oil ratios of 25:1, catalyst loadings of 1.5% w/v, and temperatures of 65 °C. Sahani et al. ( 2019 ) used a solid-base catalyst called barium cerate in the transesterification procedure to produce biodiesel from Karanja oil. To synthesize perovskite barium cerate with maximum phase purity, the calcination temperature was optimized. At 1.2 wt% catalyst, 1:19 oil-to-methanol molar ratio, 65 °C, 100 min, and 600 rpm, karanja oil methyl ester with 98.3% conversion was obtained. Kamel et al. ( 2019 ) utilized the fig leaves that had undergone calcination, KOH activation, and activation. The highest conversion to biodiesel (92.73%) was obtained from fig leaves treated with KOH under ideal conditions (2 h of heating, a 6:1 alcohol/oil molar ratio, 1% catalyst, and 400 rpm). Singh et al. ( 2023 ) produced biodiesel from Jatropha curcas oil using the transesterification technique and calcium oxide. The results of the experiment demonstrate that at a methanol/oil ratio of 12:1, 65 °C, 3 h, and a catalyst concentration of 5 wt%, a biodiesel yield of 81.6% was produced. Carbon spheres were the heterogeneous acid catalyst that Nata et al. ( 2017 ) utilized. A maximum yield of 87% was achieved at 60 °C and 1 h when WCO was used as the feedstock to make biodiesel utilizing a C–SO 3 H acid catalyst. Du et al. ( 2019 ) converted Scenedesmus quadricauda algal oil into biodiesel using a cobalt-doped CaO catalyst. Cao was obtained from eggshells and calcined at 400, 700, and 900 °C. Todorović et al. ( 2019 ) conducted research on canola oil-based potassium-supported TiO 2 for biodiesel generation. At 55 °C for 5 h, with a 6 wt% catalyst and a 54/1 methanol/oil, the highest biodiesel output of > 90% was discovered. Salinas et al. ( 2012 ) created a carbon-based MgO catalyst for castor oil transesterification utilizing the sol–gel method. With a 96.5% biodiesel output at 6 wt% catalyst loading and a 12:1 ethanol/oil ratio at 75 °C for 1 h, the MgO/UREA-800 demonstrated remarkable catalytic activity. Gardy et al. ( 2019 ) made a strong, magnetic core–shell SO 4 /Mg–Al–FeO 3 heterogeneous catalyst with the use of surface functionalization, encapsulation, and stepwise coprecipitation. Utilizing the synthesized catalyst, the transesterification reaction was carried out with the highest possible yield of 98.5% at 9:1 methanol/WCO, 95 °C, and 5 h. Table 4 highlights some of the recently published research on the use of several heterogeneous catalyst types for biodiesel synthesis, various feedstock sources, experimental setups, and biodiesel yields.
Enzyme-based catalyst
Enzyme-based catalysts are produced from living things that speed up reactions while maintaining the stability of their composition (Amini et al. 2017 ). Extracellular lipases are the enzymes that have been isolated and processed from the microbial broth. In contrast, intracellular lipase remains inside the cell or in its walls of production (Gog et al. 2012 ). One drawback of employing extracellular enzymes as catalysts is the expense and difficulty of the separation and purification procedures (Rizwanul Fattah et al. 2020 ). The efficiency of the bio-catalyzed transesterification process is influenced by the enzyme’s source and the process variables (Aransiola et al. 2014 ). Enzymatic biodiesel production also has the advantages of being simple to remove, operating at a temperature between 35 and 45 °C, producing no byproducts, and allowing catalysts to be reused (Christopher et al. 2014 ). For the transesterification of low-grade fish oil, Marín-Suárez et al. ( 2019 ) used Novozym 435 lipase; the greatest FAEE yield was 82.91 wt% after 8 h, 35 °C, an excess of ethanol, and 1% catalyst. Novozym 435 can be used for 10 continuous cycles with a maximum activity decrease of 16%. Jayaraman et al. ( 2020 ) studied used cooking oil enzymatic transesterification with the use of pancreatic lipase to make methyl ester. The best reaction conditions were discovered to be methanol as the alcohol 3:1 M ratio, 1.5% enzyme concentration (by weight of WCO), 4 h reaction duration, 60 °C, and 88% yield after numerous attempts. Fatty acid methyl ester (FAME) was produced by Choi et al. ( 2018 ) produced FAME from the oil in rice bran by just adding methanol. The 83.4% yield was reached after 12 days under ideal conditions.
Nanocatalysts in transesterification
Nanocatalysts have garnered significant interest in the production of biodiesel. Because of their special qualities, which include a large active surface area, high reusability, better catalytic efficiency, high biodiesel conversion, and sustainability, nanocatalysts can be superior to conventional catalysts (Qiu et al. 2011 ). Since they are easily removed from the final products and retain their catalytic activity even after being reused several times, nanocatalysts are widely sought (Ahmed et al. 2023 ). There are numerous ways to create nanocatalysts. Among the techniques are microwave combustion, chemical vapor deposition, impregnation, and gas condensation (Quirino et al. 2016 ; Ambat et al. 2018 ). Some of the latest works on nanocatalysts for the transesterification reaction are listed in Table 5 .
Metal-oxide nanocatalysts
The most popular nanocatalysts are those based on metal oxide, and they play a crucial role in maximizing the synthesis of biodiesel. Nanoparticles that will be employed for transesterification catalysis have been created using the oxidized forms of numerous different metals, including Mg, Zn, and Ca (Pandit et al. 2023 ). Jamil et al. ( 2021 ) created highly efficient barium oxide using catalysts made of molybdenum oxide. Optimal conditions include 12 methanol/oil, 120 min, 65 °C, and a 4.5wt% catalyst. The best yield was achieved under these conditions, which resulted in a 97.8% yield. Sahani et al. ( 2020 ) produced biodiesel with a transesterification reaction involving used cooking oil and a mixed metal oxide catalyst made of Sr–Ti. Methanol as the alcohol in an 11:1 M ratio, 1% catalyst, an 80-min reaction period, and a temperature of 65 °C with 98% FAME conversion were found to be the best reaction conditions. In a study conducted by Tayeb et al. ( 2023 ), the production of biodiesel using a CaO catalyst through the transesterification of WCO was investigated. The study determined the optimal reaction parameters to be a WCO/methanol molar ratio of 1:6, a 1% CaO nanocatalyst, a reaction temperature of 70 °C, and a reaction duration of 85 min, which resulted in a 97% biodiesel yield.
Carbon nanocatalysts
Nanocatalysts are created from carbon materials, including graphene and reduced graphene oxides (Nizami and Rehan 2018 ). Due to their diverse structural, mechanical, thermal, and biocompatibility qualities, carbon nanocatalysts are good catalysts and have advantageous applications in electrocatalytic devices such as fuel cells and other electro-processing systems. CNTs are often manufactured from graphite sheets that have been wound into cylinder forms. They have a large surface area, measure in nanometers, and are incredibly biocompatible (Rai et al. 2016 ).
Zeolite nanocatalysts
Large exterior surface areas and the hydrophobic nature of nanozeolites increase enzyme access to the substrate. Natural zeolite materials are far less frequently used in commercial industries than synthetic-based products. Commercially available synthetic zeolites such as ZSM-5, X, Y, and beta are used primarily in the production of biodiesel (Abukhadra et al. 2019 ). Using zeolites from NaY, KL, and NaZSM-5, Wu et al. ( 2013 ) produced CaO catalysts that were utilized to catalyze the transformation of methanol with soybean oil. In comparison to pure CaO, the activities of synthesized catalysts were studied. It was discovered that after being supported by zeolites, the CaO catalyst’s activity improved, with the CaO/NaY catalyst showing the greatest performance. Using the CaO/NaY catalyst, methanol-to-soybean oil 9:1 molar ratio at 65 °C with a reaction period of 3 h, and a 3% catalyst were used to produce a 95% biodiesel yield. Firouzjaee and Taghizadeh ( 2017 ) synthesized a CaO/NaY-Fe 3 O 4 nano-magnetic catalyst that was employed for the generation of biodiesel. The ideal methanol-to-oil molar ratio is 8.78, the catalyst loading is 5.19% (30% CaO loaded on the surface nanomagnetic zeolite), and the reaction period is 4 h. The maximum methyl esters obtained are 95.37%.
Distinct behavior of nanocatalysts during biodiesel production
Nanocatalysts have been widely used in biodiesel production due to their high catalytic activity, low cost, and environmental friendliness. The properties of nanocatalysts can vary depending on the preparation method, which can affect their catalytic performance. For example, the size, shape, and surface area of the catalyst particles can influence the reaction kinetics and yield of biodiesel. Recent studies have investigated the effects of different preparation methods on the properties of nanocatalysts for biodiesel production. The preparation method and calcination temperature are important factors that can affect the properties and catalytic performance of nanocatalysts for biodiesel production. Further research is needed to optimize the preparation methods and properties of nanocatalysts to improve the efficiency and sustainability of biodiesel production. We can offer general insights into the variations of nanocatalysts throughout the biodiesel production process, focusing on the following aspects.
Catalyst types: Different generations of nanocatalysts may involve distinct types of materials. For instance, first-generation nanocatalysts might include basic materials, while second- or third-generation may involve more advanced materials like metal oxides, zeolites, or other nanostructured materials.
Particle size: Advances in nanotechnology enable the control of particle size in nanocatalysts. The particle size can significantly impact catalytic activity. Smaller particle sizes may provide larger surface areas and enhanced catalytic efficiency.
Functionalization: The functionalization of nanocatalysts with specific groups or ligands can vary across generations. Functionalization can influence the catalyst’s selectivity and stability during biodiesel production.
Reusability and stability: Reusability and recovery are the two main advantages of using heterogeneous nanocatalysts in the production of biodiesel. The nanocatalyst is recovered and utilized again at each stage of these processes, which include many cycles of producing biodiesel. Nanocatalysts are often recovered via chemical means. The intended product and any byproduct may be easily and quickly recovered from the reaction mixture thanks to heterogeneous catalysts. This type of catalyst eliminates the need for a washing step. The esterification method using nanocatalysts was proposed to have several benefits, including speedier mixing of the reactants and catalyst and easy and rapid separation from the reaction mixture (Pandit et al. 2023 ).
Synthesis methods: The methods used to synthesize nanocatalysts may evolve, affecting their structure and properties. Recent advancements might include greener synthesis approaches or techniques that enhance the reproducibility of catalysts.
In addition to the aspects mentioned, the surface chemistry of nanocatalysts can also vary across generations, affecting their catalytic behavior during biodiesel production. The surface chemistry of nanocatalysts can be modified through various methods, such as surface functionalization, doping, or coating, to tune their catalytic activity, selectivity, and stability. For instance, surface functionalization with organic molecules or inorganic ions can enhance the catalyst’s selectivity for specific reactions or improve its compatibility with the reaction medium. The use of nanocatalysts in biodiesel production also presents some challenges, such as the aggregation, fouling, and leaching of active species. These issues can lead to a decrease in catalytic activity and selectivity, as well as an increase in production costs. To address these challenges, researchers are exploring various strategies, such as surface modification, stabilization techniques, and immobilization methods, to improve the stability and reusability of nanocatalysts. In summary, the distinct behavior of nanocatalysts during biodiesel production is influenced by various factors, including catalyst type, particle size, functionalization, surface chemistry, synthesis methods, and stability. The optimization of these factors can lead to more efficient, selective, and sustainable biodiesel production processes. However, further research is needed to fully understand the underlying mechanisms and to develop new generations of nanocatalysts with enhanced performance and stability.
Transesterification reaction mechanism (alcoholysis)
A large variety of exchange reactions involving oils, fats, and other reactants may be explained by the reaction mechanism. This comprises three processes: (1) transesterification, a rearrangement that yields monoglyceride, diglyceride, or other esters; (2) acidolysis, which involves exchanging fatty acids to produce specific fatty acid products; and (3) alcoholysis, which produces methyl esters in reactions with monohydric alcohols and monyl glycerol in reactions with polyhydric alcohols. Natural vegetable oils, animal fats, and food industry waste oil may all be utilized as source materials for transesterification, a process that produces biodiesel. Methanol, ethanol, propanol, butanol, and pentanol are among the alcohols that can be utilized for transesterification. Because it is a cheap, short-chain, strong polar raw material that reacts rapidly with fatty acid glycerides, methanol is the most widely used of them. Also freely soluble in methanol are base catalysts. A catalytic agent in this reaction might be an acid, base, or enzyme. Base catalysts consist of carbonate, NaOH, KOH, and potassium and sodium alkaloids. Acid catalysts might be hydrochloric, phosphoric, or sulfuric acids. The enzyme lipase is a good catalyst for the esterification of alcohols to fatty acid glycerides. Figures 7 , 8 , 9 , and 10 represent continuous reversible processes for transesterification reactions; every reaction yields a distinct type of alcohol (Kang et al. 2015 ; Sait et al. 2022 ; Li et al. 2020 ; Oyekunle et al. 2023 ).
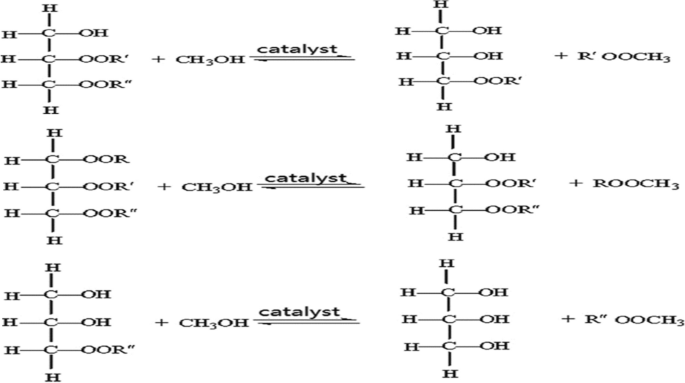
Continuous reversible processes of transesterification reactions

Acid-catalyzed alcoholysis reaction mechanism

Base-catalyzed alcoholysis reaction mechanism
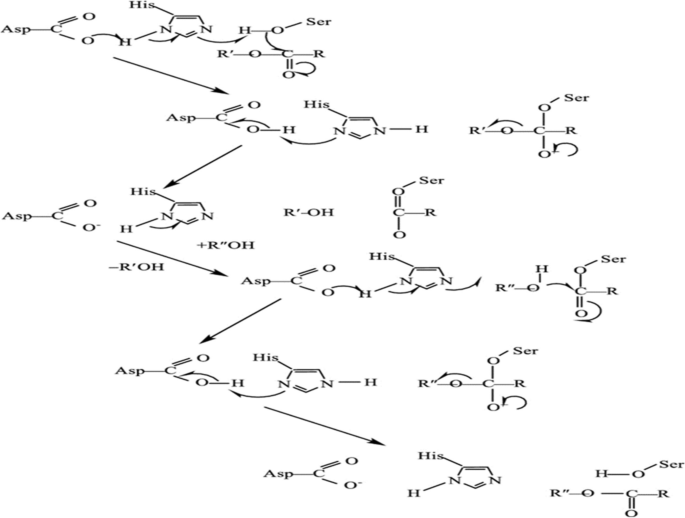
Enzyme-catalyzed alcoholysis reaction mechanism
Kinetic modeling’s significance for process optimization
Kinetic models of chemical processes are powerful tools for reactor design. The kinetic models are very helpful in choosing the best reaction conditions (temperature, pressure, mixing rate, etc.) for chemical or biochemical transformations in reactors or bioreactors. This maximizes the formation of desired products with the least material investment and financial resources. This also holds true for the many techniques used to produce biodiesel, such as homogeneous, heterogeneous, enzyme catalysis, and others. One of the most important stages in the development of chemical processes for industrial applications is thought to be carefully thought-out experimental research and the subsequent creation of a kinetic model (Trejo-Zárraga et al. 2018 ). Portha et al. ( 2012 ) were able to decrease the extra ethanol used in the transesterification reaction in a continuous mode. By adjusting the temperature of the second reactor and adding methanol in stages, they were able to enhance the system’s overall performance, as demonstrated by the results of their simulation. Using triolein as a model chemical, the authors conducted experiments and discovered that it was beneficial to convert diglyceride and monoglyceride in the second reactor and the majority of triolein in the first. Additionally, their calculations suggested that to improve reaction rates at this point, it would be prudent to raise the temperature in the second reactor. Additionally, the authors computed internal concentration profiles using a reactor model that included the kinetic model. They discovered the limiting phenomenon in the overall transformation. To get a deeper comprehension of the rates of output and the inhibitory patterns seen in the transformation scheme, a kinetic model may also be strategically employed (Firdaus et al. 2016 ). For instance, a reaction scheme for the enzymatic creation of biodiesel might consider many more reaction stages and, consequently, a greater number of parameters. This adds difficulty to the kinetic model creation process, but once this model is solved, it may be utilized to construct an enzyme-catalyzed reactor and eventually optimize the process. The use of kinetic models, which can faithfully replicate the process at various reaction conditions, is helpful in the field of research and process improvement as it offers guidelines for additional experimental work and helps eliminate potentially fruitless experimental trials. Additionally, models may be utilized to foresee how composition will affect the final product’s quality. A model might forecast, for instance, how the feedstock’s water content or FFA may impact the reaction conversion and, in turn, the biodiesel’s production and quality.
Kinetics of transesterification
Few studies have dealt with kinetic modeling; most of the heterogeneous catalysis research has been on the manufacture and utilization of catalysts. To achieve reaction conditions with inherent kinetics and minimal effects, efforts have been focused on using tiny solid particles. It has been discovered that most heterogeneous transesterifications adhere to a pseudo-first-order model. For instance, Kaur and Ali ( 2014 ) discovered that the ethanolysis of Jatropha curcas L. oil, which were catalyzed by 15-Zr/CaO-700, adhered to a pseudo-first-order rate law. The Koros-Nowak test proved that the transit impacts were insignificant. Lukić et al. ( 2014 ) also discovered a first-order reversible rate law under ideal circumstances for the transesterification of sunflower oil. Table 6 lists some kinetic modeling studies of heterogeneous transesterification.
Characterization methods for the assessment of produced biodiesels
Characterization methods for the assessment of produced biodiesel include various analytical techniques to evaluate the quality and properties of biodiesel. These methods are essential for ensuring that biodiesel meets the required standards and specifications for use as a sustainable and efficient alternative fuel source. The American Society for Testing and Materials (ASTM) is a prominent organization that provides authoritative guidelines for biodiesel testing and characterization methods.
The most common characterization methods for assessing produced biodiesel include the following.
Fatty acid methyl ester (FAME) analysis: FAME analysis is a fundamental method for biodiesel characterization, involving the determination of the fatty acid methyl ester content in biodiesel. This analysis is typically performed using gas chromatography (GC) or high-performance liquid chromatography (HPLC) to quantify individual FAME components, which provides valuable information about the biodiesel’s composition and purity.
Viscosity measurement: Viscosity is a crucial parameter for biodiesel quality assessment, as it affects the flow behavior and performance of the fuel. Dynamic viscosity measurements are commonly conducted to determine the resistance of biodiesel to flow under specific conditions, offering insights into its suitability for use in engines and transportation applications.
Oxidation stability testing: Biodiesel’s resistance to oxidation is an important characteristic that influences its shelf life and storage stability. Various methods, such as the Rancimat test and the PetroOXY test, are employed to assess the oxidation stability of biodiesel by measuring its susceptibility to oxidative degradation over time.
Cold flow properties analysis: The cold flow properties of biodiesel, including cloud point and pour point, are critical factors affecting its performance in cold weather conditions. Characterization methods such as differential scanning calorimetry (DSC) and automated cloud and pour point analyzers are utilized to determine these properties, ensuring that biodiesel remains operational at low temperatures.
Acid value determination: The acid value of biodiesel indicates its acidity level, which can impact engine components and fuel system integrity. Acid value determination involves titration methods to quantify the amount of free fatty acids present in biodiesel, enabling the assessment of its corrosiveness and potential impact on engine performance.
Calorific value measurement: Calorific value, also known as heating value, represents the energy content of biodiesel and is crucial for evaluating its combustion efficiency and heat output. Bomb calorimetry is commonly used to measure the calorific value of biodiesel, providing essential data for assessing its energy potential as a fuel source.
Sulfur content analysis: Sulfur content determination is essential for ensuring compliance with environmental regulations and assessing the environmental impact of biodiesel combustion. Techniques such as X-ray fluorescence (XRF) spectroscopy or ultraviolet fluorescence analysis are employed to measure sulfur levels in biodiesel samples.
Glycerol content quantification: Glycerol content in biodiesel must be monitored to ensure compliance with quality standards and prevent potential issues related to fuel stability and engine performance. Analytical methods like gas chromatography coupled with flame ionization detection (GC-FID) are utilized for the accurate quantification of glycerol in biodiesel products.
These characterization methods collectively provide comprehensive insights into the chemical composition, physical properties, stability, and environmental impact of produced biodiesel, supporting quality control measures and regulatory compliance within the biofuel industry.
The recent development in biodiesel production
Biodiesel, a renewable and sustainable alternative to conventional diesel fuel, has seen significant developments in recent years. These advancements have focused on improving the efficiency of biodiesel production processes, expanding feedstock options, and enhancing the overall sustainability of biodiesel as a viable energy source. One notable recent development is the use of advanced catalysts in biodiesel production. Catalysts play a crucial role in the conversion of vegetable oils or animal fats into biodiesel through a process called transesterification. Researchers have been exploring various catalysts, such as solid acid catalysts, enzyme catalysts, and heterogeneous catalysts, to improve reaction rates, reduce energy consumption, and enhance biodiesel quality. These catalysts offer advantages like higher conversion rates, milder reaction conditions, and easier separation of the catalyst from the product (Garcia-Silvera et al. 2023 ). Another significant development is the utilization of non-traditional feedstocks for biodiesel production. While conventional biodiesel feedstocks include soybean oil and rapeseed oil, researchers have been investigating alternative sources such as algae, waste cooking oil, and non-food crops like jatropha and camelina. Algae have gained attention due to their high oil content and ability to grow in various environments. The use of non-traditional feedstocks helps to reduce competition with food production and enhances the overall sustainability of biodiesel (Garg et al. 2023 ). Furthermore, efforts have been made to improve the sustainability of biodiesel production by reducing its environmental impact. This includes optimizing production processes to minimize water and energy consumption, reducing greenhouse gas emissions, and implementing waste management strategies. Additionally, researchers have been exploring the concept of “second-generation” biodiesel, which involves utilizing waste materials, such as agricultural residues and lignocellulosic biomass, to produce biodiesel. This approach not only reduces waste but also maximizes resource utilization (Makepa et al. 2023 ).
Biodiesel engine performance and emissions
Compared to petrodiesel fuel, burning biodiesel releases fewer particulates, carbon monoxide, and unburned hydrocarbons. Since biodiesel is produced using natural resources, its sulfur content is relatively low, which means that when it burns in an engine, it releases less sulfur dioxide into the atmosphere (Rayati et al. 2020 ). All biodiesels and their blends have shown the capacity to enhance gas turbine performance while lowering emissions of carbon dioxide, carbon monoxide, nitrogen oxide, and hydrocarbons under a range of operating conditions. To employ fuels in an engine, one must be aware of their characteristics for combustion. Although fossil fuel-based diesel fuel may not be entirely replaced by biodiesel, it can aid in achieving balanced energy utilization. One benefit is that biodiesel may be used in contemporary engines with little modification. Older vehicles with natural rubber gasoline lines, however, require a few modifications. Rubber fuel lines must be replaced since they will crack when used with biodiesel. On the other hand, an oil or gasoline dilution in the fuel system is possible in a modern vehicle with a DPF (diesel particulate filter). The ability of gasoline to lubricate the fuel injection system is believed to be crucial for diesel engines. The use of diesel–biodiesel mixes can thereby enhance their general lubricity. Additionally, the lower sulfur level of today’s diesel fuel could affect its lubricity because the compounds that provided lubrication are no longer present (Veza et al. 2022 ).
Techno-economic analysis
Techno-economic analysis (TEA) plays a crucial role in assessing the economic feasibility and viability of biodiesel production processes. It involves evaluating the overall costs, revenues, and profitability of biodiesel production, considering various factors such as feedstock costs, capital investment, operational expenses, and market prices. Recent studies have employed TEA to analyze and optimize biodiesel production processes, providing valuable insights for decision-making and process design. One example of TEA in biodiesel production is a study conducted by Zhang ( 2021 ), which evaluated the techno-economic performance of different feedstocks and process configurations for biodiesel production. The analysis considered factors such as feedstock availability, conversion efficiency, capital costs, operating costs, and market prices. The study highlighted the importance of feedstock selection and process optimization in achieving cost-effective biodiesel production. Another study by Tasić ( 2020 ) performed TEA for manufacturing biodiesel from used cooking oil. The analysis included the estimation of capital and operational costs, energy consumption, and environmental impacts. The study demonstrated the economic feasibility of waste cooking oil-based biodiesel production and identified critical parameters affecting the overall economics of the process. Furthermore, a study by Atabani ( 2020 ) conducted TEA for biodiesel production from microalgae. The analysis considered various scenarios, including different cultivation systems and conversion technologies. The study assessed the economic viability of microalgae-based biodiesel production, considering factors such as biomass productivity, lipid content, capital investment, and operational costs. These recent studies emphasize the importance of TEA in evaluating the economic aspects of biodiesel production. By considering a comprehensive range of factors, TEA provides valuable insights into the cost-effectiveness, profitability, and sustainability of biodiesel production processes, helping guide decision-making and process optimization.
Challenges, perspectives, and further research
The homogeneous catalyst has been thoroughly examined, and the literature has addressed several issues. However, heterogeneous catalysts are a very new field of study, and there is now a lot of research being done in this area. The literature has documented many obstacles regarding these catalysts:
It has been said that the primary issues with heterogeneous catalysts are instability, reduced reaction rate, and short catalyst life.
It has been reported that solid-base catalysts are FFA, CO 2 , and water sensitive. Through saponification, they destroy the catalyst and render it inactive.
Because water hydrolyzes the ionic group in solid acid catalysts, leaching and product contamination have been documented.
It has been documented that during enzymatic transesterification, methanol causes lipase inhibition.
In the case of nanocatalysts, good performance requires increasing the reaction time under very moderate working conditions. To attain normal reaction times, however, harsh working conditions must be used, which raises energy consumption.
Future research should pay attention to the following recommendations:
To create novel catalysts with enhanced catalytic performance, more research into waste-derived catalysts is required.
The creation of highly selective and active heterogeneous catalysts that may be used in industrial settings at a reasonable cost.
Investigating novel catalyst supports with a network of linked pores of the right size and a selected surface area.
Investigating the use of waste or biomass as a catalyst source to lower related costs and increase sustainability for solid catalysts that are sold commercially.
Improving synthesis catalyst production procedures and treatment stages to move the technology from a lab to an industrial setting.
Maintaining the high basic strength of the synthesis catalyst while improving the shape and sensitivity to FFA and water.
Additional research is needed into the manufacturing of industrial enzymatic biodiesel as a guaranteed choice for the future.
To give relevant information about the suitable catalyst, future research on the catalytic mechanism of partial catalysts should examine the features in depth.
Ways to recover and reuse nanocatalysts that are both economical and energy efficient.
Kinetic studies of the biodiesel production reaction using the synthesized catalyst should be carried out.
This extensive review delves into the various aspects of biodiesel production and its promise as a sustainable alternative for a greener energy future. The significance of feedstock selection and preparation is emphasized, with effective techniques discussed for optimizing biodiesel production efficiency and quality. Biodiesel has emerged as a versatile and promising alternative for transportation, industrial processes, and energy generation, demonstrating its potential to reduce greenhouse gas emissions and dependency on fossil fuels. The key process of transesterification is thoroughly examined, encompassing the utilization of diverse catalysts, including homogeneous, heterogeneous, enzyme based, and nanomaterials. The unique characteristics and performance of nanomaterials in transesterification are highlighted, offering prospects for enhanced efficiency and selectivity. Understanding the reaction mechanism and kinetics of transesterification is crucial for optimizing the production process. Kinetic modeling is identified as a valuable tool for process optimization, enabling better control and improved production efficiency. Methods for assessing the quality and properties of produced biodiesel are discussed, highlighting the importance of accurate characterization to meet quality standards and ensure compatibility with engine systems. Recent developments in biodiesel production showcase progress in feedstock selection, process optimization, and sustainability. However, challenges related to engine performance, emissions, and compatibility remain obstacles to wider biodiesel adoption. Future research should focus on addressing these challenges through innovative engine technologies, improved fuel formulations, and effective emission control strategies. Techno-economic analysis provides insights into the economic feasibility of biodiesel production, considering factors such as feedstock costs, process efficiency, and market demand. Ongoing analysis and assessment are essential for ensuring the commercial viability and scalability of biodiesel production. In conclusion, biodiesel presents a promising sustainable solution, but its advancement requires continuous research, development, and collaboration among academia, industry, and policymakers. Addressing challenges, pursuing further research, and implementing the recommendations outlined in this review will contribute to the widespread adoption of biodiesel as a renewable energy source, paving the way for a cleaner and more sustainable future.

Data Availability
Data available on request from the authors.
Abbas Ghazali A, Marahel F (2022) Determining the characteristics of biodiesel from a mixture of crop mustard and edible waste oil using heterogeneous calcium oxide catalyst prepared from fish bones and predicting the efficiency of biodiesel using Taguchi statistical program model. J Phys Theor Chem IAU Iran 18:37–47. https://doi.org/10.30495/JPTC.2022.21451
Article Google Scholar
Abdel-Hamid SMS, Tharwat KM, Gad AM, Asmaa AY, Sohair TA (2023) Process optimization for biodiesel production and its effect on the performance of diesel engines. Chem Eng Technol 46:694–701. https://doi.org/10.1002/ceat.202200157
Article CAS Google Scholar
Abdulrahman RK (2017) Sustainable biodiesel production from waste cooking oil and chicken fat as an alternative fuel for diesel engine. Eur Sci J 13. https://doi.org/10.19044/esj.2016.v13n3p235
Abdulsalam H (2023) Production of biodiesel from yellow oleander (Thevetin peruviana (Pers. K Schum) seed oil. Arid Zone J Basic Appl Res 2:1–9. https://doi.org/10.55639/607.201918
Abukhadra MR, Ibrahim SM, Yakout SM, Mohamed E (2019) Synthesis of Na+ trapped bentonite/zeolite-P composite as a novel catalyst for effective production of biodiesel from palm oil; Effect of ultrasonic irradiation and mechanism. Energy Convers Manag 196:739–750. https://doi.org/10.1016/j.enconman.2019.06.027
Aghel B, Mohadesi M, Ansari A, Maleki M (2019) Pilot-scale production of biodiesel from waste cooking oil using kettle limescale as a heterogeneous catalyst. Renew Energy 142:207–214. https://doi.org/10.1016/j.renene.2019.04.100
Ahmed M, Ahmad KA, Vo DVN, Mohammed Y(2023) Recent trends in sustainable biodiesel production using heterogeneous nanocatalysts: function of supports, promoters, synthesis techniques, reaction mechanism, and kinetics and thermodynamic studies. Energy Convers Manag 280. https://doi.org/10.1016/j.enconman.2023.116821
Al Hatrooshi AS, Eze VC, Harvey AP (2020) Production of biodiesel from waste shark liver oil for biofuel applications. Renew Energy 145:99–105. https://doi.org/10.1016/j.renene.2019.06.002
Alaei S, Haghighi M, Toghiani J, Rahmani Vahid B (2018) Magnetic and reusable MgO/MgFe2O4 nanocatalyst for biodiesel production from sunflower oil: influence of fuel ratio in combustion synthesis on catalytic properties and performance. Ind Crops Prod 117:322–332. https://doi.org/10.1016/j.indcrop.2018.03.015
Ali A, Li B, Lu Y, Zhao C (2019) Highly selective and low-temperature hydrothermal conversion of natural oils to fatty alcohols. Green Chem 21:3059–3064. https://doi.org/10.1039/C9GC01260E
Al-Mawaali S, Al-Balushi K, Souissi Y (2023) Sustainable biodiesel production from waste cooking oil and waste animal fats. In: Proceedings of the 8th World Congress on Civil, Structural, and Environmental Engineering. Avestia Publishing. https://doi.org/10.11159/iceptp23.145
Alptekin E, Canakci M, Sanli H (2014) Biodiesel production from vegetable oil and waste animal fats in a pilot plant. Waste Manag 34:2146–2154. https://doi.org/10.1016/j.wasman.2014.07.019
Article CAS PubMed Google Scholar
Ambat I, Srivastava V, Sillanpää M (2018) Recent advancement in biodiesel production methodologies using various feedstock: a review. Renew Sustain Energy Rev 90:356–369. https://doi.org/10.1016/j.rser.2018.03.069
Amini Z, Ilham Z, Ong HC, Hoora M, Wei HC (2017) State of the art and prospective of lipase-catalyzed transesterification reaction for biodiesel production. Energy Convers Manag 141:339–353. https://doi.org/10.1016/j.enconman.2016.09.049
Amirthavalli V, Warrier AR (2019) Production of biodiesel from waste cooking oil using MgO nanocatalyst. In: AIP Conference Proceedings. American Institute of Physics Inc. https://doi.org/10.1063/1.5113448
Amruth Maroju P, Ganesan R, Ray Dutta J (2023) Biofuel generation from food waste through immobilized enzymes on magnetic nanoparticles. Mater Today Proc 72:62–66. https://doi.org/10.1016/j.matpr.2022.05.555
Aransiola EF, Ojumu TV, Oyekola OO (2014) A review of current technology for biodiesel production: state of the art. Biomass Bioenergy 61:276–297. https://doi.org/10.1016/j.biombioe.2013.11.014
Arora R, Toor AP, Wanchoo RK (2015) Esterification of high free fatty acid rice bran oil: parametric and kinetic study. Chem Biochem Eng Q 29:617–623. https://doi.org/10.15255/CABEQ.2014.2117
Ashok A, Kennedy LJ, Vijaya JJ, Aruldoss U (2018) Optimization of biodiesel production from waste cooking oil by magnesium oxide nanocatalyst synthesized using coprecipitation method. Clean Technol Environ Policy 20:1219–1231. https://doi.org/10.1007/s10098-018-1547-x
Atabani AE (2020) Techno-economic analysis of biodiesel production from microalgae: a review. Renew Sustain Energy Rev 124:109770. https://doi.org/10.30638/eemj.2013.191
Atadashi IM, Aroua MK, Abdul Aziz AR, Sulaiman NMN (2012) The effects of water on biodiesel production and refining technologies: a review. Renew Sustain Energy Rev 16:3456–3470. https://doi.org/10.1016/j.rser.2012.03.004
Avhad MR, Marchetti JM (2015) A review on recent advancement in catalytic materials for biodiesel production. Renew Sustain Energy Rev 50:696–718. https://doi.org/10.1016/j.rser.2015.05.038
Azizian H, Kramer JKG (2005) A rapid method for the quantification of fatty acids in fats and oils with emphasis on trans fatty acids using Fourier transform near infrared spectroscopy (FT-NIR). Lipids 40:855–867. https://doi.org/10.1007/s11745-005-1448-3
Balat M (2011) Potential alternatives to edible oils for biodiesel production – a review of current work. Energy Convers Manag 52:1479–1492. https://doi.org/10.1016/j.enconman.2010.10.011
Belkhanchi H, Rouan M, Hammi M (2021) Synthesis of biodiesel by transesterification of used frying oils (Ufo) through basic homogeneous catalysts (NaOH and KOH). Biointerface Res Appl Chem 11:12858–12868. https://doi.org/10.33263/BRIAC115.1285812868
Bhatia SK, Gurav R, Choi TR (2020) Conversion of waste cooking oil into biodiesel using heterogenous catalyst derived from cork biochar. Bioresour Technol 302. https://doi.org/10.1016/j.biortech.2020.122872
Bhuana DS, Qadariyah L, Panjaitan R, Mahfud M (2020) Optimization of biodiesel production from Chlorella sp through in-situ microwave-Assisted acid-catalyzed trans-esterification. In: IOP Conference Series: Materials Science and Engineering. Institute of Physics Publishing. https://doi.org/10.1088/1757-899X/732/1/012004
Booramurthy VK, Kasimani R, Subramanian D, Pandian S (2020) Production of biodiesel from tannery waste using a stable and recyclable nano-catalyst: an optimization and kinetic study. Fuel 260. https://doi.org/10.1016/j.fuel.2019.116373
Çakırca EE, Tekin GN, İlgen O, Akın AN (2019) Catalytic activity of CaO-based catalyst in transesterification of microalgae oil with methanol. Energy Environ 30:176–187. https://doi.org/10.1177/0958305X18787317
Celante D, Schenkel JVD, de Castilhos F (2018) Biodiesel production from soybean oil and dimethyl carbonate catalyzed by potassium methoxide. Fuel 212:101–107. https://doi.org/10.1016/j.fuel.2017.10.040
Chen Y, Liu T, He H, Liang H (2018) Fe3O4/ZnMg(Al)O magnetic nanoparticles for efficient biodiesel production. Appl Organomet Chem 32:e4330. https://doi.org/10.1002/aoc.4330
Choi N, No DS, Kim H (2018) In situ lipase-catalyzed transesterification in rice bran for synthesis of fatty acid methyl ester. Ind Crops Prod 120:140–146. https://doi.org/10.1016/j.indcrop.2018.04.049
Choksi H, Pandian S, Arumugamurthi SS (2021) Production of biodiesel from high free fatty acid feedstock using heterogeneous acid catalyst derived from palm-fruit-bunch. Energy Sources, Part a: Recovery, Util Environ Eff 43:3393–3402. https://doi.org/10.1080/15567036.2019.1623953
Christopher LP, Kumar H, Zambare VP (2014) Enzymatic biodiesel: challenges and opportunities. Appl Energy 119:497–520. https://doi.org/10.1016/j.apenergy.2014.01.017
Article CAS ADS Google Scholar
da Costa JM, de Andrade Lima LRP (2021) Transesterification of cotton oil with ethanol for biodiesel using a KF/bentonite solid catalyst. Fuel 293. https://doi.org/10.1016/j.fuel.2021.120446
de Barros SS, Pessoa Junior WAG, Sá ISC (2020) Pineapple (Ananás comosus) leaves ash as a solid base catalyst for biodiesel synthesis. Bioresour Technol 312. https://doi.org/10.1016/j.biortech.2020.123569
Degfie TA, Mamo TT, Mekonnen YS (2019) Optimized biodiesel production from waste cooking oil (WCO) using calcium oxide (CaO) nano-catalyst. Sci Rep 9. https://doi.org/10.1038/s41598-019-55403-4
Demirbas A (2009) Progress and recent trends in biodiesel fuels. Energy Convers Manag 50:14–34. https://doi.org/10.1016/j.enconman.2008.09.001
Demirbas A (2008) A realistic fuel alternative for diesel engines. https://doi.org/10.1007/978-1-84628-995-8
Devaraj K, Mani Y, Rawoof SAA (2020) Feasibility of biodiesel production from waste cooking oil: lab-scale to pilot-scale analysis. Environ Sci Pollut Res 27:25828–25835. https://doi.org/10.1007/s11356-020-09068-6
Dhawane SH, Kumar T, Halder G (2018) Recent advancement and prospective of heterogeneous carbonaceous catalysts in chemical and enzymatic transformation of biodiesel. Energy Convers Manag 167:176–202. https://doi.org/10.1016/j.enconman.2018.04.073
Du W, Xu Y, Liu D, Zeng J (2004) Comparative study on lipase-catalyzed transformation of soybean oil for biodiesel production with different acyl acceptors. J Mol Catal B Enzym 30:125–129
Du L, Li Z, Ding S (2019) Synthesis and characterization of carbon-based MgO catalysts for biodiesel production from castor oil. Fuel 258:116122. https://doi.org/10.1016/j.fuel.2019.116122
Elgharbawy AS, Sadik WA, Sadek OM, Kasaby MA (2021) A review on biodiesel feedstocks and production technologies. https://doi.org/10.4067/S0717-97072021000105098
Erchamo YS, Mamo TT, Workneh GA, Mekonnen YS (2021) Improved biodiesel production from waste cooking oil with mixed methanol–ethanol using enhanced eggshell-derived CaO nano-catalyst. Sci Rep 11. https://doi.org/10.1038/s41598-021-86062-z
Fattah IMR, Masjuki HH, Kalam MA (2014) Performance and emission characteristics of a CI engine fueled with Cocos nucifera and Jatropha curcas B20 blends accompanying antioxidants. Ind Crops Prod 57:132–140
Firdaus MY, Brask J, Nielsen PM (2016) Kinetic model of biodiesel production catalyzed by free liquid lipase from Thermomyces lanuginosus. J Mol Catal B Enzym 133:55–64
Firouzjaee MH, Taghizadeh M (2017) Optimization of process variables for biodiesel production using the nanomagnetic catalyst CaO/NaY-Fe3O4. Chem Eng Technol 40:1140–1148. https://doi.org/10.1002/ceat.201600406
Freedman B, Butterfield RO, Pryde EH (1986) Transesterification kinetics of soybean oil 1. J Am Oil Chem Soc 63:1375–1380
Fukuda H, Kondo A, Noda H (2001) Biodiesel fuel production by transesterification of oils. J Biosci Bioeng 92:405–416. https://doi.org/10.1016/S1389-1723(01)80288-7
Garcia-Silvera EE, Melendez-Mogollon I, Pérez-Arias A, de la Rosa YA (2023) Enzymatic Biodiesel Production: Challenges and Opportunities. In: Congress on Research, Development, and Innovation in Renewable Energies: Selected Papers from CIDiER 2022. Springer, pp 19–37. https://doi.org/10.1007/978-3-031-26813-7_2
Gardy J, Nourafkan E, Osatiashtiani A (2019) A core-shell SO4/Mg-Al-Fe3O4 catalyst for biodiesel production. Appl Catal B 259:118093. https://doi.org/10.1016/j.apcatb.2019.118093
Garg R, Sabouni R, Ahmadipour M (2023) From waste to fuel: challenging aspects in sustainable biodiesel production from lignocellulosic biomass feedstocks and role of metal organic framework as innovative heterogeneous catalysts. Ind Crops Prod 206:117554
Ghosh N, Halder G (2022) Current progress and perspective of heterogeneous nanocatalytic transesterification towards biodiesel production from edible and inedible feedstock: a review. Energy Convers Manag 270:116292. https://doi.org/10.1016/j.enconman.2022.116292
Gog A, Roman M, Toşa M (2012) Biodiesel production using enzymatic transesterification – current state and perspectives. Renew Energy 39:10–16. https://doi.org/10.1016/j.renene.2011.08.007
Gonçalves MA, Lourenço Mares EK, da Luz PTS (2021) Biodiesel synthesis from waste cooking oil using heterogeneous acid catalyst: statistical optimization using linear regression model. J Renew Sustain Energy 13. https://doi.org/10.1063/5.0048147
Gui MM, Lee KT, Bhatia S (2008) Feasibility of edible oil vs. non-edible oil vs. waste edible oil as biodiesel feedstock. Energy 33:1646–1653. https://doi.org/10.1016/j.energy.2008.06.002
Hamed HH, Mohammed AE, Habeeb OA (2021) Biodiesel production from waste cooking oil using homogeneous catalyst. Egypt J Chem 64:2827–2832. https://doi.org/10.21608/ejchem.2021.62395.3339
Haryanto A, Gita AC, Saputra TW, Telaumbanua M (2020) First order kinetics of biodiesel synthesis using used frying oil through transesterification reaction. Aceh Int J Sci Technol 9:1–11. https://doi.org/10.13170/aijst.9.1.13297
Hayyan A, Alam MZ, Mirghani MES (2011) Reduction of high content of free fatty acid in sludge palm oil via acid catalyst for biodiesel production. Fuel Process Technol 92:920–924
Hazmi B, Rashid U, Ibrahim ML (2021) Synthesis and characterization of bifunctional magnetic nano-catalyst from rice husk for production of biodiesel. Environ Technol Innov 21. https://doi.org/10.1016/j.eti.2020.101296
Helmi M, Hemmati A (2021) Synthesis of magnetically solid base catalyst of NaOH/Chitosan-Fe3O4 for biodiesel production from waste cooking oil: optimization, kinetics and thermodynamic studies. Energy Convers Manag 248. https://doi.org/10.1016/j.enconman.2021.114807
Hoekman SK, Robbins C (2012) Review of the effects of biodiesel on NOx emissions. Fuel Process Technol 96:237–249. https://doi.org/10.1016/j.fuproc.2011.12.036
Ibrahim ML, Nik Abdul Khalil NNA, Islam A (2020) Preparation of Na2O supported CNTs nanocatalyst for efficient biodiesel production from waste-oil. Energy Convers Manag 205:112445. https://doi.org/10.1016/j.enconman.2019.112445
Jain S, Kumar N, Singh VP (2023) Transesterification of algae oil and little amount of waste cooking oil blend at low temperature in the presence of NaOH. Energies (Basel) 16. https://doi.org/10.3390/en16031293
Jain S, Sharma MP (2010) Biodiesel production from Jatropha curcas oil. Renew Sustain Energy Rev 14:3140
Jamal Y, Rabie A, Boulanger BO (2015) Determination of methanolysis rate constants for low and high fatty acid oils using heterogeneous surface reaction kinetic models. React Kinet Mech Catal 114:63–74. https://doi.org/10.1007/s11144-014-0780-5
Jamil F, Al-Riyami M, Al-Haj L (2021) Waste Balanites aegyptiaca seed oil as a potential source for biodiesel production in the presence of a novel mixed metallic oxide catalyst. Int J Energy Res 45:17189–17202. https://doi.org/10.1002/er.5609
Javidialesaadi A, Raeissi S (2013) Biodiesel production from high free fatty acid-content oils: experimental investigation of the pretreatment step. APCBEE Proc 5:474–478. https://doi.org/10.1016/j.apcbee.2013.05.080
Jayaraman J, Alagu K, Appavu P (2020) Enzymatic production of biodiesel using lipase catalyst and testing of an unmodified compression ignition engine using its blends with diesel. Renew Energy 145:399–407. https://doi.org/10.1016/j.renene.2019.06.061
Kalavathy G, Baskar G (2019) Synergism of clay with zinc oxide as nanocatalyst for production of biodiesel from marine Ulva lactuca. Bioresour Technol 281:234–238. https://doi.org/10.1016/j.biortech.2019.02.101
Kamel DA, Farag HA, Amin NK (2019) Utilization of Ficus carica leaves as a heterogeneous catalyst for production of biodiesel from waste cooking oil. Environ Sci Pollut Res 26:32804–32814. https://doi.org/10.1007/s11356-019-06424-z
Kamran E, Mashhadi H, Mohammadi A, Ghobadian B (2020) Biodiesel production from Elaeagnus angustifolia.L seed as a novel waste feedstock using potassium hydroxide catalyst. Biocatal Agric Biotechnol 25. https://doi.org/10.1016/j.bcab.2020.101578
Kang X, Guo X, You H (2015) Biodiesel development in the global scene. Energy Sources Part B 10:155–161
Kara K, Ouanji F, Lotfi EM (2018) Biodiesel production from waste fish oil with high free fatty acid content from Moroccan fish-processing industries. Egypt J Pet 27:249–255
Karmakar B, Halder G (2021) Accelerated conversion of waste cooking oil into biodiesel by injecting 2-propanol and methanol under superheated conditions: a novel approach. Energy Convers Manag 247. https://doi.org/10.1016/j.enconman.2021.114733
Karmee SK, Patria RD, Lin CSK (2015) Techno-economic evaluation of biodiesel production from waste cooking oil—a case study of Hong Kong. Int J Mol Sci 16:4362–4371. https://doi.org/10.3390/ijms16034362
Article CAS PubMed PubMed Central Google Scholar
Kasirajan R (2021) Biodiesel production by two step process from an energy source of Chrysophyllum albidum oil using homogeneous catalyst. S Afr J Chem Eng 37:161–166. https://doi.org/10.1016/j.sajce.2021.05.011
Kaur N, Ali A (2014) Kinetics and reusability of Zr/CaO as heterogeneous catalyst for the ethanolysis and methanolysis of Jatropha crucas oil. Fuel Process Technol 119:173–184
Knothe G (2010) Biodiesel and renewable diesel: a comparison. Prog Energy Combust Sci 36:364–373. https://doi.org/10.1016/j.pecs.2009.11.004
Kumar SD (2021) Biodiesel production using homogeneous, heterogeneous, and enzyme catalysts via transesterification and esterification reactions: a critical review. BioEnergy Res 15(2):935 961. https://doi.org/110.1007/s12155-021-10333-w
Kuniyil M, Shanmukha Kumar JV, Adil SF (2021) Production of biodiesel from waste cooking oil using ZnCuO/N-doped graphene nanocomposite as an efficient heterogeneous catalyst. Arab J Chem 14:102982. https://doi.org/10.1016/j.arabjc.2020.102982
Laskar IB, Rokhum L, Gupta R, Chatterjee S (2020) Zinc oxide supported silver nanoparticles as a heterogeneous catalyst for production of biodiesel from palm oil. Environ Prog Sustain Energy 39. https://doi.org/10.1002/ep.13369
Lawer-Yolar G, Dawson-Andoh B, Atta-Obeng E (2021) Synthesis of biodiesel from tall oil fatty acids by homogeneous and heterogeneous catalysis. Sustain Chem 2:206–221. https://doi.org/10.3390/suschem2010012
Lee SB, Lee JD, Hong IK (2011) Ultrasonic energy effect on vegetable oil based biodiesel synthetic process. J Ind Eng Chem 17:138–143. https://doi.org/10.1016/j.jiec.2010.12.012
Li E, Xu ZP, Rudolph V (2009) MgCoAl–LDH derived heterogeneous catalysts for the ethanol transesterification of canola oil to biodiesel. Appl Catal B 88:42–49
Li F, Jiang J, Liu P (2018) Catalytic cracking of triglycerides with a base catalyst and modification of pyrolytic oils for production of aviation fuels. Sustain Energy Fuels 2:1206–1215
Li C, Zhang A, Liu R (2020) Principle and Processing of Biodiesel Production. Industrial Oil Plant: Application Principles and Green Technologies 127–156. https://doi.org/10.1007/978-981-15-4920-5_5
Liu Y, Sotelo-Boyás R, Murata K (2011) Hydrotreatment of vegetable oils to produce bio-hydrogenated diesel and liquefied petroleum gas fuel over catalysts containing sulfided Ni–Mo and solid acids. Energy Fuels 25:4675–4685
Lukić I, Kesić Ž, Maksimović S (2013) Kinetics of sunflower and used vegetable oil methanolysis catalyzed by CaO· ZnO. Fuel 113:367–378
Lukić I, Kesić Ž, Skala D (2014) Kinetics of heterogeneous biodiesel synthesis using supported ZnO as catalyst. Chem Eng Technol 37:1879–1884
Madhuranthakam CMR, Kamyabi AMN, Almheiri GM, Elkamel A (2020) Sustainable Approach for the Production of Biodiesel from Waste Cooking Oil Using Static Mixer Technology. In: Proceedings of the International Conference on Industrial Engineering and Operations Management. https://doi.org/10.46254/AN10.20200753
Makepa DC, Chihobo CH, Musademba D (2023) Techno-economic analysis and environmental impact assessment of biodiesel production from bio-oil derived from microwave-assisted pyrolysis of pine sawdust. Heliyon 9(11). https://doi.org/10.1016/j.heliyon.2023.e22261
Manzanera M, Molina-Munoz M, Gonzalez-Lopez J (2008) Biodiesel: an alternative fuel. Recent Pat Biotechnol 2:25–34. https://doi.org/10.2174/187220808783330929
Marín-Suárez M, Méndez-Mateos D, Guadix A, Guadix EM (2019) Reuse of immobilized lipases in the transesterification of waste fish oil for the production of biodiesel. Renew Energy 140:1–8. https://doi.org/10.1016/j.renene.2019.03.035
Martchamadol J, Kumar S (2012) Thailand’s energy security indicators. Renew Sustain Energy Rev 16:6103–6122. https://doi.org/10.1016/j.rser.2012.06.021
Martínez A, Mijangos GE, Romero-Ibarra IC (2018) A novel green one-pot synthesis of biodiesel from Ricinus communis seeds by basic heterogeneous catalysis. J Clean Prod 196:340–349. https://doi.org/10.1016/j.jclepro.2018.05.241
Meher LC, Sagar DV, Naik SN (2006) Technical aspects of biodiesel production by transesterification—a review. Renew Sustain Energy Rev 10:248–268
Melero JA, Iglesias J, Morales G (2009) Heterogeneous acid catalysts for biodiesel production: current status and future challenges. Green Chem. 11:1285–1308. https://doi.org/10.1039/b902086a
Mobin S, Alam F, Chowdhury H (2022) Environmental impact of algae-based biofuel production: A review. AIP Conference Proceedings. AIP Publishing. 2681(1). https://doi.org/10.1063/5.0117093
Mofijur M, Masjuki HH, Kalam MA (2014) Comparative evaluation of performance and emission characteristics of Moringa oleifera and Palm oil based biodiesel in a diesel engine. Ind Crops Prod 53:78–84
Mohamed RM, Kadry GA, Abdel-Samad HA, Awad ME (2020) High operative heterogeneous catalyst in biodiesel production from waste cooking oil. Egypt J Pet 29:59–65. https://doi.org/10.1016/j.ejpe.2019.11.002
Mostafa F, Hassankhani A, Rafiee H (2013) Preparation and characterization of Cs/Al/Fe3O4 nanocatalysts for biodiesel production. Energy Convers Manag 71:62–68. https://doi.org/10.1016/j.enconman.2013.03.022
Mrad N, Varuvel EG, Tazerout M, Aloui F (2012) Effects of biofuel from fish oil industrial residue–diesel blends in diesel engine. Energy 44:955–963
Nata IF, Putra MD, Irawan C, Lee C-K (2017) Catalytic performance of sulfonated carbon-based solid acid catalyst on esterification of waste cooking oil for biodiesel production. J Environ Chem Eng 5:2171–2175. https://doi.org/10.1016/j.jece.2017.04.029
Naveenkumar R, Baskar G (2019) Biodiesel production from Calophyllum inophyllum oil using zinc doped calcium oxide (Plaster of Paris) nanocatalyst. Bioresour Technol 280:493–496. https://doi.org/10.1016/j.biortech.2019.02.078
Nisa K, Salwa DAA, Hasanah A, Widayat (2020) Biodiesel production from waste cooking oil by using zirconia catalyst. In: AIP Conference Proceedings. American Institute of Physics Inc 2217(1). https://doi.org/10.1063/5.0000912
Nizami A-S, Rehan M (2018) Towards nanotechnology-based biofuel industry. Biofuel Res J 5:798–799. https://doi.org/10.18331/BRJ2018.5.2.2
Noreen S, Sahar I, Masood N (2021) Thermodynamic and kinetic approach of biodiesel production from waste cooking oil using nano-catalysts. https://doi.org/10.1515/zpc-2020-1644
Oa A, Ao A, Se A (2019) Process parameter estimation of biodiesel production from waste frying oil (vegetable and palm oil) using homogeneous catalyst. J Food Process Technol 10. https://doi.org/10.35248/2157-7110.19.10.811
Oh KK, Kim YS, Yoon HH, Tae BS (2002) Pretreatment of lignocellulosic biomass using combination of ammonia recycled percolation and dilute-acid process. J Ind Eng Chem 8:64–70
CAS Google Scholar
Olubunmi BE, Karmakar B, Aderemi OM (2020) Parametric optimization by Taguchi L9 approach towards biodiesel production from restaurant waste oil using Fe-supported anthill catalyst. J Environ Chem Eng 8. https://doi.org/10.1016/j.jece.2020.104288
Ong HC, Masjuki HH, Mahlia TMI (2014) Engine performance and emissions using Jatropha curcas, Ceiba pentandra and Calophyllum inophyllum biodiesel in a CI diesel engine. Energy 69:427–445. https://doi.org/10.1016/j.energy.2014.03.035
Oyekunle DT, Barasa M, Gendy EA, Tiong SK (2023) Heterogeneous catalytic transesterification for biodiesel production: Feedstock properties, catalysts and process parameters. Process Saf Environ Prot 177(11). https://doi.org/10.1016/j.psep.2023.07.064
Pandit C, Banerjee S, Pandit S (2023) Recent advances and challenges in the utilization of nanomaterials in transesterification for biodiesel production. Heliyon 9.9(4). https://doi.org/10.1016/j.heliyon.2023.e15475
Perumal G, Mahendradas DK (2022) Biodiesel production from Bauhinia variegata seeds oil using homogeneous catalyst. Pet Sci Technol 40:857–870. https://doi.org/10.1080/10916466.2021.2008968
Portha J-F, Allain F, Coupard V (2012) Simulation and kinetic study of transesterification of triolein to biodiesel using modular reactors. Chem Eng J 207:285–298
Qiu F, Li Y, Yang D (2011) Heterogeneous solid base nanocatalyst: Preparation, characterization and application in biodiesel production. Bioresour Technol 102:4150–4156. https://doi.org/10.1016/j.biortech.2010.12.071
Quirino MR, Oliveira MJC, Keyson D (2016) Synthesis of zinc aluminate with high surface area by microwave hydrothermal method applied in the transesterification of soybean oil (biodiesel). Mater Res Bull 74:124–128. https://doi.org/10.1016/j.materresbull.2015.10.027
Rahimi T, Kahrizi D, Feyzi M, et al (2021) Catalytic performance of MgO /Fe2O3-SiO2 core-shell magnetic nanocatalyst for biodiesel production of Camelina sativa seed oil: optimization by RSM-CCD method. Ind Crops Prod 159. https://doi.org/10.1016/j.indcrop.2020.113065
Rai M, dos Santos JC, Soler MF (2016) Strategic role of nanotechnology for production of bioethanol and biodiesel. https://doi.org/10.1515/ntrev-2015-0069
Rajkumari K, Rokhum L (2020) A sustainable protocol for production of biodiesel by transesterification of soybean oil using banana trunk ash as a heterogeneous catalyst. Biomass Convers Biorefin 10:839–848. https://doi.org/10.1007/s13399-020-00647-8
Rashid U, Ahmad J, Ibrahim ML (2019) Single-pot synthesis of biodiesel using efficient sulfonated-derived tea waste-heterogeneous catalyst. Materials 12. https://doi.org/10.3390/ma12142293
Rayati M, Rajabi Islami H, Shamsaie Mehrgan M (2020) Light intensity improves growth, lipid productivity, and fatty acid profile of Chlorococcum oleofaciens (Chlorophyceae) for biodiesel production. Bioenergy Res 13:1235–1245
Rizwanul Fattah IM, Ong HC, Mahlia TMI (2020) State of the art of catalysts for biodiesel production. Front Energy Res 8. https://doi.org/10.3389/fenrg.2020.00101
Roy T, Ágarwal AK, Sharma YC (2021) A cleaner route of biodiesel production from waste frying oil using novel potassium tin oxide catalyst: a smart liquid-waste management. Waste Manag 135:243–255. https://doi.org/10.1016/j.wasman.2021.08.046
Ruhul AM, Kalam MA, Masjuki HH et al (2015) State of the art of biodiesel production processes: a review of the heterogeneous catalyst. RSC Adv 5:101023–101044. https://doi.org/10.1039/C5RA09862A
Sadaf S, Iqbal J, Ullah I et al (2018) Biodiesel production from waste cooking oil: an efficient technique to convert waste into biodiesel. Sustain Cities Soc 41:220–226. https://doi.org/10.1016/j.scs.2018.05.037
Saeed A, Hanif MA, Hanif A, et al (2021) Production of biodiesel from spirogyra elongata, a common freshwater green algae with high oil content. Sustainability (Switzerland) 13. https://doi.org/10.3390/su132212737
Sahani S, Roy T, Sharma YC (2020) Smart waste management of waste cooking oil for large scale high quality biodiesel production using Sr-Ti mixed metal oxide as solid catalyst: optimization and E-metrics studies. Waste Manag 108:189–201. https://doi.org/10.1016/j.wasman.2020.04.036
Sahani S, Roy T, Chandra Sharma Y (2019) Clean and efficient production of biodiesel using barium cerate as a heterogeneous catalyst for the biodiesel production; kinetics and thermodynamic study. J Clean Prod 237. https://doi.org/10.1016/j.jclepro.2019.117699
Sait HH, Hussain A, Bassyouni M, Ali I, Kanthasamy R, Ayodele BV, Elhenawy Y (2022) Hydrogen-rich syngas and biochar production by non-catalytic valorization of date palm seeds. Energies 15(8):2727
Salinas D, Araya P, Guerrero S (2012) Study of potassium-supported TiO2 catalysts for the production of biodiesel. Appl Catal B 117–118:260–267. https://doi.org/10.1016/j.apcatb.2012.01.016
Sayed A, El-Gharbawy AA (2016) Production of biodiesel from non edible vegetable oil
Seffati K, Honarvar B, Esmaeili H, Esfandiari N (2019) Enhanced biodiesel production from chicken fat using CaO/CuFe2O4 nanocatalyst and its combination with diesel to improve fuel properties. Fuel 235:1238–1244. https://doi.org/10.1016/j.fuel.2018.08.118
Sharma YC, Singh B (2009) Development of biodiesel: current scenario. Renew Sustain Energy Rev 13:1646–1651. https://doi.org/10.1016/j.rser.2008.08.009
Siddiqua S (2015) Transesterification of palm oil to biodiesel and optimization of production conditions i.e. methanol, sodium hydroxide and temperature. J Energy Nat Resour 4:45. https://doi.org/10.11648/j.jenr.20150403.12
Singh SP, Singh D (2010) Biodiesel production through the use of different sources and characterization of oils and their esters as the substitute of diesel: a review. Renew Sustain Energy Rev 14:200–216. https://doi.org/10.1016/j.rser.2009.07.017
Singh A, Choudhary AK, Sinha S (2023) Analysis of vibrations in a diesel engine produced by Jatropha biodiesel using heterogeneous catalyst. Energy Environ 34:407–428. https://doi.org/10.1177/0958305X211063935
Singh A, Hoque ME, Chuan YL (2011) Biodiesel from low cost feedstocks: The effects of process parameters on the biodiesel yield. Biomass and Bioenergy 35(4):1582–1587. https://doi.org/10.1016/j.biombioe.2010.12.024
Sousa LL, Lucena IL, Fernandes FAN (2010) Transesterification of castor oil: effect of the acid value and neutralization of the oil with glycerol. Fuel Process Technol 91:194–196. https://doi.org/10.1016/J.FUPROC.2009.09.016
Srivastava N, Srivastava M, Gupta VK (2018) Recent development on sustainable biodiesel production using sewage sludge. 3 Biotech 8:1–11. https://doi.org/10.1007/s13205-018-1264-5
Stamenković OS, Veljković VB, Todorović ZB (2010) Modeling the kinetics of calcium hydroxide catalyzed methanolysis of sunflower oil. Bioresour Technol 101:4423–4430. https://doi.org/10.1016/j.biortech.2010.01.109
Suzihaque MUH, Alwi H, Kalthum Ibrahim U (2022) Biodiesel production from waste cooking oil: a brief review. Mater Today Proc 63:S490–S495. https://doi.org/10.1016/j.matpr.2022.04.527
Tacias-Pascacio VG, Torrestiana-Sánchez B, Dal Magro L (2019) Comparison of acid, basic and enzymatic catalysis on the production of biodiesel after RSM optimization. Renew Energy 135:1–9. https://doi.org/10.1016/j.renene.2018.11.107
Takase M, Kipkoech R, Miller DL, Buami EK (2023) Optimisation of the reaction conditions for biodiesel from Parkia biglobosa oil via transesterification with heterogeneous clay base catalyst. Fuel Commun 15:100089. https://doi.org/10.1016/j.jfueco.2023.100089
Talebian-Kiakalaieh A, Amin NAS, Zarei A, Noshadi I (2013) Transesterification of waste cooking oil by heteropoly acid (HPA) catalyst: optimization and kinetic model. Appl Energy 102:283–292. https://doi.org/10.1016/j.apenergy.2012.07.018
Tasić MB (2020) Techno-economic analysis of biodiesel production from waste cooking oil. Environ Sci Pollut Res 27:4183–4192. https://doi.org/10.1007/s41247-018-0050-7
Tasić MB, Miladinović MR, Stamenković OS (2015) Kinetic modeling of sunflower oil methanolysis catalyzed by calcium-based catalysts. Chem Eng Technol 38:1550–1556. https://doi.org/10.1002/ceat.201500076
Tayeb AM, Abdel-Hamid SMS, Osman RM, Farouk SM (2023) Optimization of biodiesel production from waste cooking oil using nano calcium oxide catalyst. Chem Eng Technol. https://doi.org/10.1002/ceat.202300238
Thangaraj B, Solomon PR, Muniyandi B (2019) Catalysis in biodiesel production—a review. Clean Energy 3:2–23. https://doi.org/10.1093/ce/zky020
Todorović ZB, Troter DZ, Đokić-Stojanović DR (2019) Optimization of CaO-catalyzed sunflower oil methanolysis with crude biodiesel as a cosolvent. Fuel 237:903–910. https://doi.org/10.1016/j.fuel.2018.10.056
Touqeer T, Mumtaz MW, Mukhtar H (2020) Fe3O4-PDA-lipase as surface functionalized nano biocatalyst for the production of biodiesel using waste cooking oil as feedstock: characterization and process optimization. Energies (Basel) 13. https://doi.org/10.3390/en13010177
Trejo-Zárraga F, de Jesús Hernández-Loyo F, Chavarría-Hernández JC, Sotelo-Boyás R (2018) Kinetics of transesterification processes for biodiesel production. Biofuels-State Dev 149–179. https://doi.org/10.5772/intechopen.75927
Tripathi G, Dubey P, Yadav P (2023) Advancement in biodiesel production methodologies using different feedstock. In: Wastewater Resource Recovery and Biological Methods. Springer, pp 323–341. https://doi.org/10.1007/978-3-031-40198-5_15
Um B-H, Kim Y-S (2009) Review: A chance for Korea to advance algal-biodiesel technology. J Ind Eng Chem 15:1–7. https://doi.org/10.1016/j.jiec.2008.08.002
Veljković VB, Stamenković OS, Todorović ZB (2009) Kinetics of sunflower oil methanolysis catalyzed by calcium oxide. Fuel 88:1554–1562. https://doi.org/10.1016/j.fuel.2009.02.013
Veza I, Zainuddin Z, Tamaldin N (2022) Effect of palm oil biodiesel blends (B10 and B20) on physical and mechanical properties of nitrile rubber elastomer. Results Eng 16:100787. https://doi.org/10.1016/j.rineng.2022.100787
Wu H, Zhang J, Wei Q (2013) Transesterification of soybean oil to biodiesel using zeolite supported CaO as strong base catalysts. Fuel Process Technol 109:13–18. https://doi.org/10.1016/j.fuproc.2012.09.032
Xie W, Wang J (2014) Enzymatic production of biodiesel from soybean oil by using immobilized lipase on Fe3O4/poly (styrene-methacrylic acid) magnetic microsphere as a biocatalyst. Energy Fuels 28:2624–2631. https://doi.org/10.1021/ef500131s
Zhang Y (2021) techno-economic analysis of biodiesel production from different feedstocks. Energy Convers Manag 235:113948. https://doi.org/10.3390/en14051473
Download references
Open access funding provided by The Science, Technology & Innovation Funding Authority (STDF) in cooperation with The Egyptian Knowledge Bank (EKB).
Author information
Authors and affiliations.
Chemical Engineering Department, Egyptian Academy for Engineering and Advanced Technology (EA&EAT), affiliated to the Ministry of Military Production, Km. 3 Cairo Belbeis Desert Rd., Cairo Governorate, 3066, Egypt
Sabah Mohamed Farouk & Shereen M. S. Abdel-Hamid
Faculty of Engineering, Minia University, Misr Aswan Agricultural Rd., EL MAHATTA, Menia Governorate, 2431384, Egypt
Aghareed M. Tayeb
Chemical Engineering and Pilot Plant Department, National Research Centre (NRC), 33 El Bohouth St., Dokki, 12622, Giza Governorate, Egypt
Randa M. Osman
You can also search for this author in PubMed Google Scholar
Contributions
All authors contributed to the study conception and design. Data collection and analysis were performed by Sabah Mohamed Farouk, Aghareed M. Tayeb, Shereen M. S. Abdel-Hamid, and Randa M. Osman.
Corresponding author
Correspondence to Sabah Mohamed Farouk .
Ethics declarations
Ethical approval and consent to participate.
Not applicable.
Consent for publication
Competing interests.
The authors declare no competing interests.
Additional information
Responsible Editor: Ta Yeong Wu
Publisher's Note
Springer Nature remains neutral with regard to jurisdictional claims in published maps and institutional affiliations.
Rights and permissions
Open Access This article is licensed under a Creative Commons Attribution 4.0 International License, which permits use, sharing, adaptation, distribution and reproduction in any medium or format, as long as you give appropriate credit to the original author(s) and the source, provide a link to the Creative Commons licence, and indicate if changes were made. The images or other third party material in this article are included in the article's Creative Commons licence, unless indicated otherwise in a credit line to the material. If material is not included in the article's Creative Commons licence and your intended use is not permitted by statutory regulation or exceeds the permitted use, you will need to obtain permission directly from the copyright holder. To view a copy of this licence, visit http://creativecommons.org/licenses/by/4.0/ .
Reprints and permissions
About this article
Farouk, S.M., Tayeb, A.M., Abdel-Hamid, S.M.S. et al. Recent advances in transesterification for sustainable biodiesel production, challenges, and prospects: a comprehensive review. Environ Sci Pollut Res 31 , 12722–12747 (2024). https://doi.org/10.1007/s11356-024-32027-4
Download citation
Received : 16 August 2023
Accepted : 12 January 2024
Published : 23 January 2024
Issue Date : February 2024
DOI : https://doi.org/10.1007/s11356-024-32027-4
Share this article
Anyone you share the following link with will be able to read this content:
Sorry, a shareable link is not currently available for this article.
Provided by the Springer Nature SharedIt content-sharing initiative
- Sustainable biodiesel
- Nanocatalysts
- Transesterification
- Characterization
- Challenges and prospect
- Find a journal
- Publish with us
- Track your research
Information
- Author Services
Initiatives
You are accessing a machine-readable page. In order to be human-readable, please install an RSS reader.
All articles published by MDPI are made immediately available worldwide under an open access license. No special permission is required to reuse all or part of the article published by MDPI, including figures and tables. For articles published under an open access Creative Common CC BY license, any part of the article may be reused without permission provided that the original article is clearly cited. For more information, please refer to https://www.mdpi.com/openaccess .
Feature papers represent the most advanced research with significant potential for high impact in the field. A Feature Paper should be a substantial original Article that involves several techniques or approaches, provides an outlook for future research directions and describes possible research applications.
Feature papers are submitted upon individual invitation or recommendation by the scientific editors and must receive positive feedback from the reviewers.
Editor’s Choice articles are based on recommendations by the scientific editors of MDPI journals from around the world. Editors select a small number of articles recently published in the journal that they believe will be particularly interesting to readers, or important in the respective research area. The aim is to provide a snapshot of some of the most exciting work published in the various research areas of the journal.
Original Submission Date Received: .
- Active Journals
- Find a Journal
- Journal Proposal
- Proceedings Series
- For Authors
- For Reviewers
- For Editors
- For Librarians
- For Publishers
- For Societies
- For Conference Organizers
- Open Access Policy
- Institutional Open Access Program
- Special Issues Guidelines
- Editorial Process
- Research and Publication Ethics
- Article Processing Charges
- Testimonials
- Preprints.org
- SciProfiles
- Encyclopedia
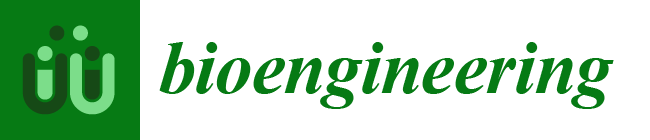
Article Menu

- Subscribe SciFeed
- Recommended Articles
- PubMed/Medline
- Google Scholar
- on Google Scholar
- Table of Contents
Find support for a specific problem in the support section of our website.
Please let us know what you think of our products and services.
Visit our dedicated information section to learn more about MDPI.
JSmol Viewer
Sustainable feedstocks and challenges in biodiesel production: an advanced bibliometric analysis.
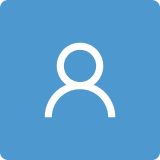
Graphical Abstract
1. Introduction
2. materials and methods, 2.1. data sources, 2.2. data analysis, 3. results and discussion, 3.1. bibliometric analysis, 3.1.1. publications: general results, 3.1.2. distribution of scientific journals, 3.1.3. distribution by country and institution, 3.1.4. most cited articles, 3.1.5. research areas, 3.2. feedstocks for biodiesel production, 3.2.1. classification of feedstocks, 3.2.2. sewage sludge, 3.2.3. microalgae, 3.2.4. waste cooking oil, 4. trendy research topics, 4.1. quantitative analysis of frequent keywords, 4.2. research areas, 4.2.1. research fields, 4.2.2. emerging trends, 4.2.3. two key insights, 5. conclusions.
- One of the key raw materials highlighted in this work, sewage sludge, has received little attention in the literature, considering that the number of cited articles is still small and despite the economic and sustainability advantages cited above. This is mostly due to the fact it is still a recent research theme and that raw materials that have been explored for longer are more attractive owing to the vast knowledge repertoire.
- Waste cooking oil and microalgae are the raw materials of most significant presence in the academic outputs analyzed. These are feedstocks that have been extensively reported on in the literature, mainly due to their long-standing reputation in the area. However, there has been a noticeable reduction in the volume of cited articles over the years.
- China, Malaysia, and India are the countries with the greatest research outputs relating to feedstocks for biodiesel production. It can be concluded, therefore, that Asia shows a great interest in this area. One factor that can explain this interest may be the sheer number of people concentrated in this region, since China, India, and Malaysia account for more than 35% of the world’s total population.
- For a more specific analysis of this research, keywords collected by the CiteSpace program were used. From a systematic verification of the terms, it was possible to observe that the main research topics in this area include oleaginous yeasts, ionic liquids, process design, oil extraction, enzymatic biodiesel production, and calcium oxide.
- Two broad perspectives related to this research area have been emphasized. The first is the generalized view of the articles that engage in the topic of feedstocks for biodiesel production. It is concluded that there is a great deal of discussion regarding the economy versus sustainability dilemma, and researchers have sought practical solutions to the problems that arise from this. The second perspective concerns the recent emergence of academic interest in studying sewage sludge for biodiesel production. It is understood that this area will be further explored in the near future due to the solution that this raw material represents to the conundrum above.
Author Contributions
Institutional review board statement, informed consent statement, data availability statement, acknowledgments, conflicts of interest.
- De Man, R.; German, L. Certifying the sustainability of biofuels: Promise and reality. Energy Policy 2017 , 109 , 871–883. [ Google Scholar ] [ CrossRef ]
- Adnan, A.I.; Ong, M.Y.; Nomanbhay, S.; Chew, K.W.; Show, P.L. Technologies for biogas upgrading to biomethane: A review. Bioengineering 2019 , 6 , 92. [ Google Scholar ] [ CrossRef ] [ PubMed ] [ Green Version ]
- Soleymani, M.; Rosentrater, K. Techno-economic analysis of biofuel production from macroalgae (seaweed). Bioengineering 2017 , 4 , 92. [ Google Scholar ] [ CrossRef ] [ Green Version ]
- Kang, A.; Lee, T.S. Converting sugars to biofuels: Ethanol and beyond. Bioengineering 2015 , 2 , 184–203. [ Google Scholar ] [ CrossRef ] [ Green Version ]
- Awogbemi, O.; Kallon, D.V.V. Application of tubular reactor technologies for the acceleration of biodiesel production. Bioengineering 2022 , 9 , 347. [ Google Scholar ] [ CrossRef ] [ PubMed ]
- Rai, A.K.; Al Makishah, N.H.; Wen, Z.; Gupta, G.; Pandit, S.; Prasad, R. Recent developments in lignocellulosic biofuels, a renewable source of bioenergy. Fermentation 2022 , 8 , 161. [ Google Scholar ] [ CrossRef ]
- Zhao, X.; Wei, L.; Cheng, S.; Julson, J. Review of heterogeneous catalysts for catalytically upgrading vegetable oils into hydrocarbon biofuels. Catalysts 2017 , 7 , 83. [ Google Scholar ] [ CrossRef ] [ Green Version ]
- Moreira, K.S.; Moura Júnior, L.S.; Monteiro, R.R.C.; de Oliveira, A.L.B.; Valle, C.P.; Freire, T.M.; Fechine, P.B.A.; de Souza, M.C.M.; Fernandez-Lorente, G.; Guisan, J.M.; et al. Optimization of the Production of Enzymatic Biodiesel from Residual Babassu Oil ( Orbignya sp.) via RSM. Catalysts 2020 , 10 , 414. [ Google Scholar ] [ CrossRef ] [ Green Version ]
- de Oliveira, A.L.B.; Cavalcante, F.T.T.; da Silva Moreira, K.; de Castro Monteiro, R.R.; Rocha, T.G.; da Silva Souza, J.E.; da Fonseca, A.M.; Lopes, A.A.S.; Guimarões, A.P.; de Lima, R.K.C.; et al. Lipases immobilized onto nanomaterials as biocatalysts in biodiesel production: Scientific context, challenges, and opportunities. Rev. Virtual Química 2021 , 13 , 875–891. [ Google Scholar ] [ CrossRef ]
- Monteiro, R.R.C.; Arana-Peña, S.; da Rocha, T.N.; Miranda, L.P.; Berenguer-Murcia, Á.; Tardioli, P.W.; dos Santos, J.C.S.; Fernandez-Lafuente, R. Liquid lipase preparations designed for industrial production of biodiesel. Is it really an optimal solution? Renew. Energy 2021 , 164 , 1566–1587. [ Google Scholar ] [ CrossRef ]
- Cavalcante, F.T.T.; Neto, F.S.; Rafael de Aguiar Falcão, I.; Erick da Silva Souza, J.; de Moura Junior, L.S.; da Silva Sousa, P.; Rocha, T.G.; de Sousa, I.G.; de Lima Gomes, P.H.; de Souza, M.C.M.; et al. Opportunities for improving biodiesel production via lipase catalysis. Fuel 2021 , 288 , 119577. [ Google Scholar ] [ CrossRef ]
- Rocha, T.G.; de L Gomes, P.H.; de Souza, M.C.M.; Monteiro, R.R.C.; dos Santos, J.C.S. Lipase cocktail for optimized biodiesel production of free fatty acids from residual chicken oil. Catal. Lett. 2021 , 151 , 1155–1166. [ Google Scholar ] [ CrossRef ]
- Mota, G.F.; de Sousa, I.G.; de Oliveira, A.L.B.; Cavalcante, A.L.G.; da Silva Moreira, K.; Cavalcante, F.T.T.; da Silva Souza, J.E.; de Aguiar Falcão, Í.R.; Rocha, T.G.; Valério, R.B.R.; et al. Biodiesel production from microalgae using lipase-based catalysts: Current challenges and prospects. Algal Res. 2022 , 62 , 102616. [ Google Scholar ] [ CrossRef ]
- Athar, M.; Zaidi, S. A review of the feedstocks, catalysts, and intensification techniques for sustainable biodiesel production. J. Environ. Chem. Eng. 2020 , 8 , 104523. [ Google Scholar ] [ CrossRef ]
- Ma, X.; Gao, M.; Gao, Z.; Wang, J.; Zhang, M.; Ma, Y.; Wang, Q. Past, current, and future research on microalga-derived biodiesel: A critical review and bibliometric analysis. Environ. Sci. Pollut. Res. 2018 , 25 , 10596–10610. [ Google Scholar ] [ CrossRef ]
- Jiang, W.; Aishan, T.; Halik, Ü.; Wei, Z.; Wumaier, M. A Bibliometric and Visualized Analysis of Research Progress and Trends on Decay and Cavity Trees in Forest Ecosystem over 20 Years: An Application of the CiteSpace Software. Forests 2022 , 13 , 1437. [ Google Scholar ] [ CrossRef ]
- Li, N.; Han, R.; Lu, X. Bibliometric analysis of research trends on solid waste reuse and recycling during 1992–2016. Resour. Conserv. Recycl. 2018 , 130 , 109–117. [ Google Scholar ] [ CrossRef ]
- Donthu, N.; Kumar, S.; Mukherjee, D.; Pandey, N.; Lim, W.M. How to conduct a bibliometric analysis: An overview and guidelines. J. Bus. Res. 2021 , 133 , 285–296. [ Google Scholar ] [ CrossRef ]
- Kargbo, D.M. Biodiesel production from municipal sewage sludges. Energy Fuels 2010 , 24 , 2791–2794. [ Google Scholar ] [ CrossRef ]
- Callegari, A.; Hlavinek, P.; Capodaglio, A.G. Production of energy (biodiesel) and recovery of materials (biochar) from pyrolysis of urban waste sludge. Rev. Ambiente Água 2018 , 13 , 1. [ Google Scholar ] [ CrossRef ]
- Tamala, J.K.; Maramag, E.I.; Simeon, K.A.; Ignacio, J.J. A bibliometric analysis of sustainable oil and gas production research using VOSviewer. Clean. Eng. Technol. 2022 , 7 , 100437. [ Google Scholar ] [ CrossRef ]
- Sweileh, W.M.; Al-Jabi, S.W.; AbuTaha, A.S.; Zyoud, S.H.; Anayah, F.M.A.; Sawalha, A.F. Bibliometric analysis of worldwide scientific literature in mobile—Health: 2006–2016. BMC Med. Inform. Decis. Mak. 2017 , 17 , 72. [ Google Scholar ] [ CrossRef ] [ PubMed ] [ Green Version ]
- Chen, C. CiteSpace II: Detecting and visualizing emerging trends and transient patterns in scientific literature. J. Am. Soc. Inf. Sci. Technol. 2006 , 57 , 359–377. [ Google Scholar ] [ CrossRef ] [ Green Version ]
- Veillette, M.; Chamoumi, M.; Nikiema, J.; Faucheux, N.; Heitz, M. Production of biodiesel from microalgae. In Advances in Chemical Engineering ; InTech: Rijeka, Croatia, 2012. [ Google Scholar ]
- Shah, S.H. Sustainable biodiesel production. In Encyclopedia of Renewable and Sustainable Materials ; Elsevier: Amsterdam, The Netherlands, 2020; pp. 347–355. [ Google Scholar ]
- Muthukumaran, C.; Sharmila, G.; Manojkumar, N.; Gnanaprakasam, A.; Sivakumar, V.M. Optimization and kinetic modeling of biodiesel production. In Encyclopedia of Renewable and Sustainable Materials ; Elsevier: Amsterdam, The Netherlands, 2020; pp. 193–201. [ Google Scholar ]
- Mata, T.M.; Martins, A.A.; Caetano, N.S. Microalgae for biodiesel production and other applications: A review. Renew. Sustain. Energy Rev. 2010 , 14 , 217–232. [ Google Scholar ] [ CrossRef ] [ Green Version ]
- Aslam, M.M.; Khan, A.A.; Cheema, H.M.N.; Hanif, M.A.; Azeem, M.W.; Azmat, M.A. Novel mutant camelina and jatropha as valuable feedstocks for biodiesel production. Sci. Rep. 2020 , 10 , 21868. [ Google Scholar ] [ CrossRef ]
- Ghani, N.; Iqbal, J.; Sadaf, S.; Nawaz Bhatti, H.; Asgher, M. Comparison of photo-esterification capability of bismuth vanadate with reduced graphene oxide bismuth vanadate (RGO/BiVO 4 ) composite for biodiesel production from high free fatty acid containing non-edible oil. ChemistrySelect 2020 , 5 , 9245–9253. [ Google Scholar ] [ CrossRef ]
- Mureed, K.; Kanwal, S.; Hussain, A.; Noureen, S.; Hussain, S.; Ahmad, S.; Ahmad, M.; Waqas, R. Biodiesel production from algae grown on food industry wastewater. Environ. Monit. Assess. 2018 , 190 , 271. [ Google Scholar ] [ CrossRef ]
- da Silva Dutra, L.; Costa Cerqueira Pinto, M.; Cipolatti, E.P.; Aguieiras, E.C.G.; Manoel, E.A.; Greco-Duarte, J.; Guimarães Freire, D.M.; Pinto, J.C. How the biodiesel from immobilized enzymes production is going on: An advanced bibliometric evaluation of global research. Renew. Sustain. Energy Rev. 2022 , 153 , 111765. [ Google Scholar ] [ CrossRef ]
- Mahlia, T.M.I.; Syazmi, Z.A.H.S.; Mofijur, M.; Abas, A.E.P.; Bilad, M.R.; Ong, H.C.; Silitonga, A.S. Patent landscape review on biodiesel production: Technology updates. Renew. Sustain. Energy Rev. 2020 , 118 , 109526. [ Google Scholar ] [ CrossRef ]
- Atabani, A.E.; Silitonga, A.S.; Ong, H.C.; Mahlia, T.M.I.; Masjuki, H.H.; Badruddin, I.A.; Fayaz, H. Non-edible vegetable oils: A critical evaluation of oil extraction, fatty acid compositions, biodiesel production, characteristics, engine performance and emissions production. Renew. Sustain. Energy Rev. 2013 , 18 , 211–245. [ Google Scholar ] [ CrossRef ]
- Ahmad, A.L.; Yasin, N.H.M.; Derek, C.J.C.; Lim, J.K. Microalgae as a sustainable energy source for biodiesel production: A review. Renew. Sustain. Energy Rev. 2011 , 15 , 584–593. [ Google Scholar ] [ CrossRef ]
- Halim, R.; Danquah, M.K.; Webley, P.A. Extraction of oil from microalgae for biodiesel production: A review. Biotechnol. Adv. 2012 , 30 , 709–732. [ Google Scholar ] [ CrossRef ] [ PubMed ]
- Tan, T.; Lu, J.; Nie, K.; Deng, L.; Wang, F. Biodiesel production with immobilized lipase: A review. Biotechnol. Adv. 2010 , 28 , 628–634. [ Google Scholar ] [ CrossRef ] [ PubMed ]
- Karmakar, A.; Karmakar, S.; Mukherjee, S. Properties of various plants and animals feedstocks for biodiesel production. Bioresour. Technol. 2010 , 101 , 7201–7210. [ Google Scholar ] [ CrossRef ] [ PubMed ]
- Aransiola, E.F.; Ojumu, T.V.; Oyekola, O.O.; Madzimbamuto, T.F.; Ikhu-Omoregbe, D.I.O. A review of current technology for biodiesel production: State of the art. Biomass Bioenergy 2014 , 61 , 276–297. [ Google Scholar ] [ CrossRef ]
- Koh, M.Y.; Ghazi, T.I.M. A review of biodiesel production from Jatropha curcas L. oil. Renew. Sustain. Energy Rev. 2011 , 15 , 2240–2251. [ Google Scholar ] [ CrossRef ]
- Wahlen, B.D.; Willis, R.M.; Seefeldt, L.C. Biodiesel production by simultaneous extraction and conversion of total lipids from microalgae, cyanobacteria, and wild mixed-cultures. Bioresour. Technol. 2011 , 102 , 2724–2730. [ Google Scholar ] [ CrossRef ]
- Atadashi, I.M.; Aroua, M.K.; Abdul Aziz, A.R.; Sulaiman, N.M.N. The effects of catalysts in biodiesel production: A review. J. Ind. Eng. Chem. 2013 , 19 , 14–26. [ Google Scholar ] [ CrossRef ]
- Martín, C.; Moure, A.; Martín, G.; Carrillo, E.; Domínguez, H.; Parajó, J.C. Fractional characterisation of jatropha, neem, moringa, trisperma, castor and candlenut seeds as potential feedstocks for biodiesel production in Cuba. Biomass Bioenergy 2010 , 34 , 533–538. [ Google Scholar ] [ CrossRef ]
- Srivastava, N.; Srivastava, M.; Gupta, V.K.; Manikanta, A.; Mishra, K.; Singh, S.; Singh, S.; Ramteke, P.W.; Mishra, P.K. Recent development on sustainable biodiesel production using sewage sludge. 3 Biotech 2018 , 8 , 245. [ Google Scholar ] [ CrossRef ]
- Mohiddin, M.N.B.; Tan, Y.H.; Seow, Y.X.; Kansedo, J.; Mubarak, N.M.; Abdullah, M.O.; Chan, Y.S.; Khalid, M. Evaluation on feedstock, technologies, catalyst and reactor for sustainable biodiesel production: A review. J. Ind. Eng. Chem. 2021 , 98 , 60–81. [ Google Scholar ] [ CrossRef ]
- Banković-Ilić, I.B.; Stamenković, O.S.; Veljković, V.B. Biodiesel production from non-edible plant oils. Renew. Sustain. Energy Rev. 2012 , 16 , 3621–3647. [ Google Scholar ] [ CrossRef ]
- Gardy, J.; Osatiashtiani, A.; Céspedes, O.; Hassanpour, A.; Lai, X.; Lee, A.F.; Wilson, K.; Rehan, M. A magnetically separable SO 4 /Fe-Al-TiO 2 solid acid catalyst for biodiesel production from waste cooking oil. Appl. Catal. B Environ. 2018 , 234 , 268–278. [ Google Scholar ] [ CrossRef ] [ Green Version ]
- Kwon, E.E.; Kim, S.; Jeon, Y.J.; Yi, H. Biodiesel production from sewage sludge: New paradigm for mining energy from municipal hazardous material. Environ. Sci. Technol. 2012 , 46 , 10222–10228. [ Google Scholar ] [ CrossRef ] [ PubMed ]
- Olkiewicz, M.; Plechkova, N.V.; Fabregat, A.; Stüber, F.; Fortuny, A.; Font, J.; Bengoa, C. Efficient extraction of lipids from primary sewage sludge using ionic liquids for biodiesel production. Sep. Purif. Technol. 2015 , 153 , 118–125. [ Google Scholar ] [ CrossRef ]
- Liu, X.; Zhu, F.; Zhang, R.; Zhao, L.; Qi, J. Recent progress on biodiesel production from municipal sewage sludge. Renew. Sustain. Energy Rev. 2021 , 135 , 110260. [ Google Scholar ] [ CrossRef ]
- Chang, Z.; Long, G.; Zhou, J.L.; Ma, C. Valorization of sewage sludge in the fabrication of construction and building materials: A review. Resour. Conserv. Recycl. 2020 , 154 , 104606. [ Google Scholar ] [ CrossRef ]
- Kumar, M.; Ghosh, P.; Khosla, K.; Thakur, I.S. Biodiesel production from municipal secondary sludge. Bioresour. Technol. 2016 , 216 , 165–171. [ Google Scholar ] [ CrossRef ]
- Kacprzak, M.; Neczaj, E.; Fijałkowski, K.; Grobelak, A.; Grosser, A.; Worwag, M.; Rorat, A.; Brattebo, H.; Almås, Å.; Singh, B.R. Sewage sludge disposal strategies for sustainable development. Environ. Res. 2017 , 156 , 39–46. [ Google Scholar ] [ CrossRef ]
- Arazo, R.O.; de Luna, M.D.G.; Capareda, S.C. Assessing biodiesel production from sewage sludge-derived bio-oil. Biocatal. Agric. Biotechnol. 2017 , 10 , 189–196. [ Google Scholar ] [ CrossRef ]
- Balasubramanian, S.; Tyagi, R.D. Value-added bio-products from sewage sludge. In Current Developments in Biotechnology and Bioengineering ; Elsevier: Amsterdam, The Netherlands, 2017; pp. 27–42. [ Google Scholar ]
- Wu, X.; Zhu, F.; Qi, J.; Zhao, L. Biodiesel production from sewage sludge by using alkali catalyst catalyze. Procedia Environ. Sci. 2016 , 31 , 26–30. [ Google Scholar ] [ CrossRef ] [ Green Version ]
- Navia, R.; Mittelbach, M. Could sewage sludge be considered a source of waste lipids for biodiesel production? Waste Manag. Res. 2012 , 30 , 873–874. [ Google Scholar ] [ CrossRef ] [ PubMed ]
- Branco-Vieira, M.; Mata, T.M.; Martins, A.A.; Freitas, M.A.V.; Caetano, N.S. Economic analysis of microalgae biodiesel production in a small-scale facility. Energy Rep. 2020 , 6 , 325–332. [ Google Scholar ] [ CrossRef ]
- Reza Talaghat, M.; Mokhtari, S.; Saadat, M. Modeling and optimization of biodiesel production from microalgae in a batch reactor. Fuel 2020 , 280 , 118578. [ Google Scholar ] [ CrossRef ]
- Mubarak, M.; Shaija, A.; Suchithra, T. Flocculation: An effective way to harvest microalgae for biodiesel production. J. Environ. Chem. Eng. 2019 , 7 , 103221. [ Google Scholar ] [ CrossRef ]
- Dev Sarkar, R.; Singh, H.B.; Chandra Kalita, M. Enhanced lipid accumulation in microalgae through nanoparticle-mediated approach, for biodiesel production: A mini-review. Heliyon 2021 , 7 , e08057. [ Google Scholar ] [ CrossRef ] [ PubMed ]
- Enwereuzoh, U.; Harding, K.; Low, M. Microalgae cultivation using nutrients in fish farm effluent for biodiesel production. S. Afr. J. Chem. Eng. 2021 , 37 , 46–52. [ Google Scholar ] [ CrossRef ]
- Amenaghawon, A.N.; Obahiagbon, K.; Isesele, V.; Usman, F. Optimized biodiesel production from waste cooking oil using a functionalized bio-based heterogeneous catalyst. Clean. Eng. Technol. 2022 , 8 , 100501. [ Google Scholar ] [ CrossRef ]
- Suzihaque, M.U.H.; Syazwina, N.; Alwi, H.; Ibrahim, U.K.; Abdullah, S.; Haron, N. A sustainability study of the processing of kitchen waste as a potential source of biofuel: Biodiesel production from waste cooking oil (WCO). Mater. Today Proc. 2022 , 63 , S484–S489. [ Google Scholar ] [ CrossRef ]
- Falowo, O.A.; Oladipo, B.; Taiwo, A.E.; Olaiya, A.T.; Oyekola, O.O.; Betiku, E. Green heterogeneous base catalyst from ripe and unripe plantain peels mixture for the transesterification of waste cooking oil. Chem. Eng. J. Adv. 2022 , 10 , 100293. [ Google Scholar ] [ CrossRef ]
- Cordero-Ravelo, V.; Schallenberg-Rodriguez, J. Biodiesel production as a solution to waste cooking oil (WCO) disposal. Will any type of WCO do for a transesterification process? A quality assessment. J. Environ. Manag. 2018 , 228 , 117–129. [ Google Scholar ] [ CrossRef ] [ PubMed ]
- Chen, C.; Chitose, A.; Kusadokoro, M.; Nie, H.; Xu, W.; Yang, F.; Yang, S. Sustainability and challenges in biodiesel production from waste cooking oil: An advanced bibliometric analysis. Energy Rep. 2021 , 7 , 4022–4034. [ Google Scholar ] [ CrossRef ]
- Aghbashlo, M.; Tabatabaei, M.; Amid, S.; Hosseinzadeh-Bandbafha, H.; Khoshnevisan, B.; Kianian, G. Life cycle assessment analysis of an ultrasound-assisted system converting waste cooking oil into biodiesel. Renew. Energy 2020 , 151 , 1352–1364. [ Google Scholar ] [ CrossRef ]
- Gouran, A.; Aghel, B.; Nasirmanesh, F. Biodiesel production from waste cooking oil using wheat bran ash as a sustainable biomass. Fuel 2021 , 295 , 120542. [ Google Scholar ] [ CrossRef ]
- Rezania, S.; Oryani, B.; Park, J.; Hashemi, B.; Yadav, K.K.; Kwon, E.E.; Hur, J.; Cho, J. Review on transesterification of non-edible sources for biodiesel production with a focus on economic aspects, fuel properties and by-product applications. Energy Convers. Manag. 2019 , 201 , 112155. [ Google Scholar ] [ CrossRef ]
- Ferrero, G.O.; Sánchez Faba, E.M.; Rickert, A.A.; Eimer, G.A. Alternatives to rethink tomorrow: Biodiesel production from residual and non-edible oils using biocatalyst technology. Renew. Energy 2020 , 150 , 128–135. [ Google Scholar ] [ CrossRef ]
- Patel, N.K.; Shah, S.N. Biodiesel from plant oils. In Food, Energy, and Water ; Elsevier: Amsterdam, The Netherlands, 2015; pp. 277–307. [ Google Scholar ]
- Wang, H.; Zhang, W.; Zhang, Y.; Xu, J. A bibliometric review on stability and reinforcement of special soil subgrade based on CiteSpace. J. Traffic Transp. Eng. Engl. Ed. 2022 , 9 , 223–243. [ Google Scholar ] [ CrossRef ]
- Wilks, D.S. Cluster Analysis. In International Geophysics ; Elsevier: Amsterdam, The Netherlands, 2011; Volume 100, pp. 603–616. [ Google Scholar ] [ CrossRef ]
- Chattopadhyay, A.; Mitra, M.; Maiti, M.K. Recent advances in lipid metabolic engineering of oleaginous yeasts. Biotechnol. Adv. 2021 , 53 , 107722. [ Google Scholar ] [ CrossRef ]
- Chtourou, H.; Dahmen, I.; Jebali, A.; Karray, F.; Hassairi, I.; Abdelkafi, S.; Ayadi, H.; Sayadi, S.; Dhouib, A. Characterization of Amphora sp., a newly isolated diatom wild strain, potentially usable for biodiesel production. Bioprocess Biosyst. Eng. 2015 , 38 , 1381–1392. [ Google Scholar ] [ CrossRef ]
- El-Sheekh, M.; Abomohra, A.E.-F.; Eladel, H.; Battah, M.; Mohammed, S. Screening of different species of scenedesmus isolated from Egyptian freshwater habitats for biodiesel production. Renew. Energy 2018 , 129 , 114–120. [ Google Scholar ] [ CrossRef ]
- Abomohra, A.E.-F.; El-Naggar, A.H.; Baeshen, A.A. Potential of macroalgae for biodiesel production: Screening and evaluation studies. J. Biosci. Bioeng. 2018 , 125 , 231–237. [ Google Scholar ] [ CrossRef ] [ PubMed ]
- Kosiński, S.; Rykowska, I.; Gonsior, M.; Krzyżanowski, P. Ionic liquids as antistatic additives for polymer composites—A review. Polym. Test. 2022 , 112 , 107649. [ Google Scholar ] [ CrossRef ]
- Mansir, N.; Teo, S.H.; Rashid, U.; Saiman, M.I.; Tan, Y.P.; Alsultan, G.A.; Taufiq-Yap, Y.H. Modified waste egg shell derived bifunctional catalyst for biodiesel production from high FFA waste cooking oil. A review. Renew. Sustain. Energy Rev. 2018 , 82 , 3645–3655. [ Google Scholar ] [ CrossRef ]
- Zhang, J.; Chen, S.; Yang, R.; Yan, Y. Biodiesel production from vegetable oil using heterogenous acid and alkali catalyst. Fuel 2010 , 89 , 2939–2944. [ Google Scholar ] [ CrossRef ]
- Petchsoongsakul, N.; Ngaosuwan, K.; Kiatkittipong, W.; Aiouache, F.; Assabumrungrat, S. Process design of biodiesel production: Hybridization of ester-and transesterification in a single reactive distillation. Energy Convers. Manag. 2017 , 153 , 493–503. [ Google Scholar ] [ CrossRef ]
- Zeng, D.; Li, R.; Wang, B.; Xu, J.; Fang, T. A review of transesterification from low-grade feedstocks for biodiesel production with supercritical methanol. Russ. J. Appl. Chem. 2014 , 87 , 1176–1183. [ Google Scholar ] [ CrossRef ]
- Soares, D.; Pinto, A.F.; Gonçalves, A.G.; Mitchell, D.A.; Krieger, N. Biodiesel production from soybean soapstock acid oil by hydrolysis in subcritical water followed by lipase-catalyzed esterification using a fermented solid in a packed-bed reactor. Biochem. Eng. J. 2013 , 81 , 15–23. [ Google Scholar ] [ CrossRef ]
- Jain, S.; Sharma, M.P. Biodiesel Production from Jatropha curcas Oil. Renew. Sustain. Energy Rev. 2010 , 14 , 3140–3147. [ Google Scholar ] [ CrossRef ]
- Gonfa Keneni, Y.; Mario Marchetti, J. Oil extraction from plant seeds for biodiesel production. AIMS Energy 2017 , 5 , 316–340. [ Google Scholar ] [ CrossRef ]
- Silitonga, A.S.; Masjuki, H.H.; Ong, H.C.; Yusaf, T.; Kusumo, F.; Mahlia, T.M.I. Synthesis and optimization of hevea brasiliensis and ricinus communis as feedstock for biodiesel production: A comparative study. Ind. Crops Prod. 2016 , 85 , 274–286. [ Google Scholar ] [ CrossRef ]
- Atabani, A.E.; César, A.d.S. Calophyllum inophyllum L.—A prospective non-edible biodiesel feedstock. Study of biodiesel production, properties, fatty acid composition, blending and engine performance. Renew. Sustain. Energy Rev. 2014 , 37 , 644–655. [ Google Scholar ] [ CrossRef ]
- Adachi, D.; Koda, R.; Hama, S.; Yamada, R.; Nakashima, K.; Ogino, C.; Kondo, A. An integrative process model of enzymatic biodiesel production through ethanol fermentation of brown rice followed by lipase-catalyzed ethanolysis in a water-containing system. Enzym. Microb. Technol. 2013 , 52 , 118–122. [ Google Scholar ] [ CrossRef ]
- Lokman, I.M.; Rashid, U.; Yunus, R.; Taufiq-Yap, Y.H. Carbohydrate-derived solid acid catalysts for biodiesel production from low-cost feedstocks: A review. Catal. Rev. 2014 , 56 , 187–219. [ Google Scholar ] [ CrossRef ]
- Zul, N.A.; Ganesan, S.; Hamidon, T.S.; Oh, W.-D.; Hussin, M.H. A review on the utilization of calcium oxide as a base catalyst in biodiesel production. J. Environ. Chem. Eng. 2021 , 9 , 105741. [ Google Scholar ] [ CrossRef ]
- Mazaheri, H.; Ong, H.C.; Amini, Z.; Masjuki, H.H.; Mofijur, M.; Su, C.H.; Anjum Badruddin, I.; Khan, T.M.Y. An overview of biodiesel production via calcium oxide based catalysts: Current state and perspective. Energies 2021 , 14 , 3950. [ Google Scholar ] [ CrossRef ]
- Peñarrubia Fernandez, I.A.; Liu, D.-H.; Zhao, J. LCA studies comparing alkaline and immobilized enzyme catalyst processes for biodiesel production under brazilian conditions. Resour. Conserv. Recycl. 2017 , 119 , 117–127. [ Google Scholar ] [ CrossRef ]
- Moser, B.R.; Vaughn, S.F. Efficacy of fatty acid profile as a tool for screening feedstocks for biodiesel production. Biomass Bioenergy 2012 , 37 , 31–41. [ Google Scholar ] [ CrossRef ]
- Chen, C. CiteSpace: A Practical Guide for Mapping Scientific Literature ; Nova Science: New York, NY, USA, 2016. [ Google Scholar ]
- Maheshwari, P.; Haider, M.B.; Yusuf, M.; Klemeš, J.J.; Bokhari, A.; Beg, M.; Al-Othman, A.; Kumar, R.; Jaiswal, A.K. A review on latest trends in cleaner biodiesel production: Role of feedstock, production methods, and catalysts. J. Clean. Prod. 2022 , 355 , 131588. [ Google Scholar ] [ CrossRef ]
- Ambat, I.; Srivastava, V.; Sillanpää, M. Recent advancement in biodiesel production methodologies using various feedstock: A review. Renew. Sustain. Energy Rev. 2018 , 90 , 356–369. [ Google Scholar ] [ CrossRef ]
- Bergmann, J.; Tupinambá, D.; Costa, O.Y.; Almeida, J.R.; Barreto, C.; Quirino, B. Biodiesel production in brazil and alternative biomass feedstocks. Renew. Sustain. Energy Rev. 2013 , 21 , 411–420. [ Google Scholar ] [ CrossRef ]
Click here to enlarge figure
Rank | Journal | C | IF | NP | NC | AC | P |
---|---|---|---|---|---|---|---|
1 | Renewable & sustainable energy reviews | USA | 14.982 | 33 | 8250 | 250 | 9.9% |
2 | Bioresource technology | NL | 9.642 | 16 | 1785 | 111.5 | 4.8% |
3 | Energy conversion and management | EN | 9.709 | 13 | 789 | 61.4 | 3.9% |
4 | Biomass & bioenergy | EN | 5.061 | 6 | 750 | 125 | 1.8% |
5 | Fuel | EN | 6.609 | 18 | 729 | 40.5 | 5.4% |
6 | Biofuels, bioproducts & biorefining—Biofpr | EN | 4.102 | 5 | 151 | 30.2 | 1.5% |
7 | Journal of environmental chemical engineering | EN | 5.909 | 4 | 63 | 15.7 | 1.2% |
8 | Catalysts | CH | 4.146 | 6 | 59 | 9.8 | 1.8% |
9 | Biofuels-UK | EN | 2.956 | 4 | 57 | 14.2 | 1.2% |
10 | Environmental chemistry letters | GER | 9.027 | 4 | 53 | 13.2 | 1.2% |
Rank | Country | NP | NC | AC | Total Link Strength | AC |
---|---|---|---|---|---|---|
1 | China | 53 | 2555 | 48.2 | 169 | 250 |
2 | Malaysia | 48 | 3715 | 77.4 | 353 | 111.5 |
3 | India | 48 | 2190 | 45.6 | 252 | 61.4 |
4 | United States of America | 33 | 2030 | 61.5 | 116 | 125 |
5 | Thailand | 17 | 164 | 9.6 | 55 | 40.5 |
6 | Indonesia | 16 | 1309 | 81.8 | 134 | 30.2 |
7 | Brazil | 15 | 531 | 35.4 | 51 | 15.7 |
8 | Italy | 13 | 164 | 12.6 | 42 | 9.8 |
9 | Serbia | 12 | 920 | 76.6 | 82 | 14.2 |
10 | Saudi Arabia | 12 | 492 | 41.0 | 100 | 13.2 |
Rank | Article Title | Authors | Year Published | Citations |
---|---|---|---|---|
1 | Microalgae for biodiesel production and other applications: A review [ ]. | Mata, Teresa M.; Martins, Antonio A.; Caetano, Nidia. S. | 2010 | 3162 |
2 | Non-edible vegetable oils: A critical evaluation of oil extraction, fatty acid compositions, biodiesel production, characteristics, engine performance, and emissions production [ ]. | Atabani, A. E.; Silitonga, A. S.; Ong, H. C.; Mahlia, T. M. I.; Masjuki, H. H.; Badruddin, Irfan Anjum; Fayaz, H. | 2013 | 645 |
3 | Microalgae as a sustainable energy source for biodiesel production: A review [ ]. | Ahmad, A. L.; Yasin, N. H. Mat; Derek, C. J. C.; Lim, J. K. | 2011 | 590 |
4 | Extraction of oil from microalgae for biodiesel production: A review [ ]. | Halim, Ronald; Danquah, Michael K.; Webley, Paul A. | 2012 | 586 |
5 | Biodiesel production with immobilized lipase: A review [ ]. | Tan, Tianwei; Lu, Jike; Nie, Kaili; Deng, Li; Wang, Fang | 2010 | 457 |
6 | Properties of various plants and animal feedstocks for biodiesel production [ ]. | Karmakar, Aninidita; Karmakar, Subrata; Mukherjee, Souti | 2010 | 421 |
7 | A review of current technology for biodiesel production: State of the art [ ]. | Aransiola, E. F.; Ojumu, T. V.; Oyekola, O. O.; Madzimbamuto, T. F.; Ikhu-Omoregbe, D. I. O. | 2014 | 368 |
8 | A review of biodiesel production from jatropha curcas L. oil [ ]. | Koh, May Ying; Ghazi, Tinia Idaty Mohd | 2011 | 365 |
9 | Biodiesel production by simultaneous extraction and conversion of total lipids from microalgae, cyanobacteria, and wild mixed-cultures [ ]. | Wahlen, Bradley D.; Willis, Robert M.; Seefeldt, Lance C. | 2011 | 319 |
10 | The effects of catalysts in biodiesel production: A review [ ]. | Atadashi, I. M.; Aroua, M. K.; Aziz, A. R. Abdul; Sulaiman, N. M. N. | 2013 | 308 |
Rank | Keyword | Frequency | TLS | Rank | Keyword | Frequency | TLS |
---|---|---|---|---|---|---|---|
1 | biodiesel | 225 | 978 | 13 | performance | 31 | 170 |
2 | transesterification | 145 | 714 | 14 | fuel production | 31 | 183 |
3 | waste cooking oil | 91 | 475 | 15 | lipase | 27 | 136 |
4 | optimization | 62 | 323 | 16 | Jatropha curcas | 26 | 130 |
5 | feedstocks | 61 | 285 | 17 | heterogeneous catalyst | 26 | 137 |
6 | soybean oil | 60 | 336 | 18 | free fatty acids | 25 | 158 |
7 | esterification | 50 | 272 | 19 | seed oil | 24 | 133 |
8 | vegetable oil | 47 | 252 | 20 | extraction | 22 | 100 |
9 | oil | 40 | 149 | 21 | in situ transesterification | 20 | 104 |
10 | microalgae | 40 | 189 | 22 | rapeseed oil | 20 | 111 |
11 | palm oil | 35 | 205 | 23 | vegetable oil | 20 | 111 |
12 | fuel | 31 | 159 | 24 | kinetics | 20 | 127 |
CID | Label | NS | Mean | Top Five Terms | Representative Articles |
---|---|---|---|---|---|
#0 | oleaginous yeast | 70 | 2015 | oleaginous yeast; lipid extraction; wild strain; Egyptian freshwater habitat; different species. | (CHTOUROU, 2015) and (EL-SHEEKH, 2018) |
#1 | ionic liquid | 63 | 2014 | ionic liquid; ultrasound-assisted transesterification; using calcium oxide catalyst; economic variable; acyl acceptor. | (ZHANG, 2010) and (MANSIR, 2018) |
#2 | process design | 54 | 2013 | process design; heterogeneous catalyst; subcritical water; packed bed reactor; soybean soap stock acid oil. | (ZENG, 2014) and (SOARES, 2013) |
#3 | oil extraction | 47 | 2014 | oil extraction; plant seed; Ricinus communis; Hevea brasiliensis; Calophyllum inophyllum L. | (KENENI, 2017) and (SILITONGA, 2016) |
#4 | enzymatic biodiesel production | 42 | 2013 | enzymatic biodiesel production; low-cost feedstock; carbohydrate-derived solid acid catalyst; brown rice; ethanol fermentation. | (ADACHI, 2016) and (LOKMAN, 2014) |
#5 | calcium oxide | 27 | 2016 | calcium oxide; current state; technological progress; different type; LCA studies. | (MAZAHERI, 2021) and (FERNANDEZ, PENARRUBIA, 2017) |
MDPI stays neutral with regard to jurisdictional claims in published maps and institutional affiliations. |
Share and Cite
Sales, M.B.; Borges, P.T.; Ribeiro Filho, M.N.; Miranda da Silva, L.R.; Castro, A.P.; Sanders Lopes, A.A.; Chaves de Lima, R.K.; de Sousa Rios, M.A.; Santos, J.C.S.d. Sustainable Feedstocks and Challenges in Biodiesel Production: An Advanced Bibliometric Analysis. Bioengineering 2022 , 9 , 539. https://doi.org/10.3390/bioengineering9100539
Sales MB, Borges PT, Ribeiro Filho MN, Miranda da Silva LR, Castro AP, Sanders Lopes AA, Chaves de Lima RK, de Sousa Rios MA, Santos JCSd. Sustainable Feedstocks and Challenges in Biodiesel Production: An Advanced Bibliometric Analysis. Bioengineering . 2022; 9(10):539. https://doi.org/10.3390/bioengineering9100539
Sales, Misael B., Pedro T. Borges, Manoel Nazareno Ribeiro Filho, Lizandra Régia Miranda da Silva, Alyne P. Castro, Ada Amelia Sanders Lopes, Rita Karolinny Chaves de Lima, Maria Alexsandra de Sousa Rios, and José C. S. dos Santos. 2022. "Sustainable Feedstocks and Challenges in Biodiesel Production: An Advanced Bibliometric Analysis" Bioengineering 9, no. 10: 539. https://doi.org/10.3390/bioengineering9100539
Article Metrics
Article access statistics, further information, mdpi initiatives, follow mdpi.
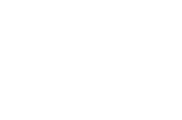
Subscribe to receive issue release notifications and newsletters from MDPI journals
Thank you for visiting nature.com. You are using a browser version with limited support for CSS. To obtain the best experience, we recommend you use a more up to date browser (or turn off compatibility mode in Internet Explorer). In the meantime, to ensure continued support, we are displaying the site without styles and JavaScript.
- View all journals
Biodiesel articles from across Nature Portfolio
Latest research and reviews.
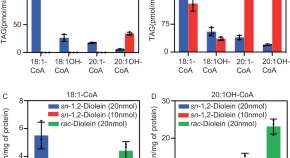
Identification of triacylglycerol remodeling mechanism to synthesize unusual fatty acid containing oils
Triacylglycerol remodeling in Physaria fendleri changes the seed oil fatty acid composition after initial synthesis to overcome metabolic bottlenecks in the accumulation of valuable unusual fatty acids. This process enhances designer oil engineering.
- Prasad Parchuri
- Sajina Bhandari
- Philip D. Bates
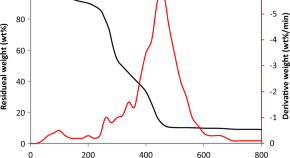
Experimental investigation on utilization of Sesbania grandiflora residues through thermochemical conversion process for the production of value added chemicals and biofuels
- Kedri Janardhana
- C. Sowmya Dhanalakshmi
- Melvin Victor De Poures
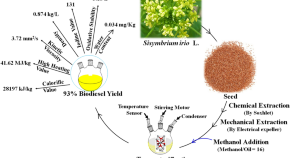
Biodiesel production from Sisymbrium irio as a potential novel biomass waste feedstock using homemade titania catalyst
- Hammad Ahmad Jan
- Ahmed I. Osman
- David W. Rooney
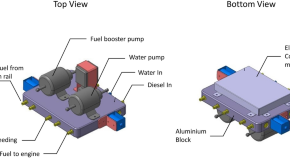
Engine performance and emissions evaluation of surfactant-free B30 biodiesel–diesel/water emulsion as alternative fuel
- Mohamad Qayyum Mohd Tamam
- Md Reashed Tasvir Omi
- Eiji Kinoshita
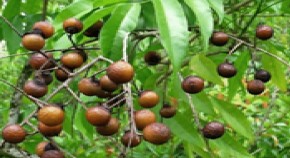
Experimental evaluation of soapberry seed oil biodiesel performance in CRDI diesel engine
- Mohammed Owais Ahmed Sajjad
- T. R. Praveenkumar
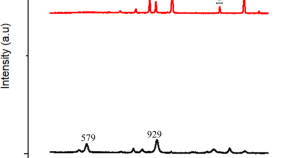
Maximizing biodiesel production from waste cooking oil with lime-based zinc-doped CaO using response surface methodology
- Mebrhit Gebreyohanes Weldeslase
- Natei Ermias Benti
- Yedilfana Setarge Mekonnen
News and Comment
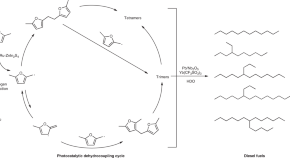
One catalyst, two fuels
Photocatalytic H 2 production using semiconductors is a promising approach to store solar energy as a chemical fuel, but the oxidizing power of the excited holes is often wasted. Now, holes are harnessed in a dehydrocoupling strategy that simultaneously produces H 2 and diesel fuel precursors from biomass-derived molecules.
- Guanqun Han
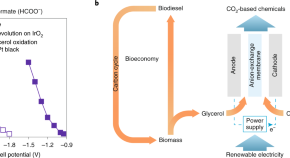
Converting two wastes to value
A significant portion of the energy required to electrochemically reduce CO 2 to fuels and chemicals is typically consumed by the accompanying oxygen evolution reaction. Now, researchers show that alternative oxidative reactions using biodiesel-waste could improve the economics and emission profiles of this process.
- André Bardow
- Matthias Wessling
Quick links
- Explore articles by subject
- Guide to authors
- Editorial policies


IMAGES
VIDEO