Einstein's Theory of Gravitation
Our modern understanding of gravity comes from Albert Einstein’s theory of general relativity, which stands as one of the best-tested theories in science. General relativity predicted many phenomena years before they were observed, including black holes , gravitational waves , gravitational lensing , the expansion of the universe , and the different rates clocks run in a gravitational field. Today, researchers continue to test the theory’s predictions for a better understanding of how gravity works.
Center for Astrophysics | Harvard & Smithsonian astrophysicists research the predictions of general relativity in many ways:
Capturing the first image of a supermassive black hole using the Event Horizon Telescope (EHT). This image of the black hole at the center of the nearby galaxy M87 reveals how gravitation affects the matter in orbit and the light that material emits, providing a novel test of general relativity in a regime where gravity is very strong. CfA Plays Central Role In Capturing Landmark Black Hole Image
Using gravitational lensing to search for the earliest galaxies in the universe. While they’re too faint to be seen directly, closer-by galaxies and clusters sometimes magnify their light, allowing us to learn about the ancestors of the Milky Way and other modern galaxies. Discovering Distant Radio Galaxies via Gravitational Lensing
Reconstructing the location of most of the mass in the universe using gravitational lensing. Next-generation observatories like the Large Synoptic Survey Telescope (LSST) will provide a census of millions of galaxies from their gravitational distortions. Mapping Dark Matter
Performing follow-up observations of gravitational wave events, to confirm the nature of the source. Collisions between neutron stars produce a lot of light in the form of short duration gamma ray bursts in addition to gravitational waves. Astronomers observed such a collision in 2017 using Dark Energy Camera on the Blanco Telescope in Chile, providing complementary data to the observation from LIGO. Astronomers See Light Show Associated With Gravitational Waves
Studying gravitational wave sources that aren’t visible to LIGO, but will be to future gravitational observatories. Using visible light telescopes, astronomers have observed that white dwarf binaries are relatively common in the galaxy, and some of them are in sufficiently tight orbits to be emitters of gravitational waves. One pair in particular orbits every 12.75 minutes, which will make it the strongest source for the future Laser Interferometer Space Antenna (LISA). Space-Warping White Dwarfs Produce Gravitational Waves
Determining whether black holes are actually what GR predicts. While GR is very clear that black holes exist, alternative theories propose different objects that behave in different ways. The challenge is that black holes appear very small in our telescopes, so it’s hard to observe their behavior. However, researchers have ruled out a number of alternative explanations, based on many observations of black holes. Do Stars Fall Quietly into Black Holes, or Crash into Something Utterly Unknown?
Testing general relativity’s prediction about the shape of a black hole. The Event Horizon Telescope is designed to take a picture of the “shadow” of the Milky Way’s supermassive black hole, which is the dark region through which no light passes. The shape of this shadow is predicted by GR, so the EHT will provide the first precision measurement of a fundamental property of a black hole. Event Horizon Telescope Reveals Magnetic Fields at Milky Way's Central Black Hole

A Century of Relativity
Albert Einstein published his full theory of general relativity in 1915, followed by a flurry of research papers by Einstein and others exploring the predictions of the theory. In general relativity (GR), concentrations of mass and energy curve the structure of spacetime, affecting the motion of anything passing near — including light. The theory explained the anomalous orbit of Mercury, but the first major triumph came in 1919 when Arthur Eddington and his colleagues measured the influence of the Sun’s gravity on light from stars during a total solar eclipse.
Physicists made many exotic predictions using general relativity. The bending of light around the Sun is small, but researchers realized the effect would be much larger for galaxies, to the point where gravity would form images of more distant objects — the phenomenon now called gravitational lensing. GR also predicted the existence of black holes: objects with gravity so intense that nothing getting too close can escape again, not even light.
General relativity showed that gravitation has a speed, which is the same as the speed of light. Catastrophic events like collisions between black holes or neutron stars produce gravitational waves. Researchers finally detected these waves in 2015 using the Laser Interferometer Gravitational Observatory (LIGO), a sensitive laboratory that took decades to develop.
For many aspects of astronomy — the motion of planets around stars, the structure of galaxies, etc. — researchers don’t need to use general relativity. However, in places where gravity is strong, and to describe the structure of the universe itself, GR is necessary. For that reason, researchers continue to use GR and probe its limits.
Black holes are extremely common in the universe. Stellar-mass black holes, the remnants of massive stars that exploded, are sometimes the source of powerful X-ray emissions when they are in binary systems with stars. In addition, nearly every galaxy harbors a supermassive black hole at its center, some of which produce powerful jets of matter visible from across the universe. GR is essential to understanding how these objects become so bright, as well as studying how black holes form and grow. The Event Horizon Telescope (EHT) is a world-spanning array of observatories that captured the first image of a supermassive black hole, providing a new arena for testing GR’s predictions.
Gravitational waves are a new branch of astronomy, providing a complementary way to study astrophysical systems to the standard light-based observations. Researchers use GR to provide “templates” of many possible gravitational wave signals, which is how they identify the source and its properties. Gravitational wave astronomy combines with light-based astronomy to characterize some of the most extreme events in the cosmos: collisions of black holes and neutron stars .
Astronomers use gravitational lensing to locate some of the earliest galaxies in the universe, which are too faint to be seen without the magnification provided by gravity. In addition, the distortion created by lensing allows researchers to study dark matter , and map the structure of the universe on the largest scales.
Not long after Einstein published GR, researchers realized the theory predicts that the universe changes in time. Observations in the 1920s found that prediction was true: the universe is expanding, with galaxies moving away from each other. Using GR, cosmologists found the cosmos had a beginning, and was once hotter and denser than it is today. GR provides the mathematical framework for describing the structure and evolution of the universe from its beginnings 13.8 billion years ago, and into the future.
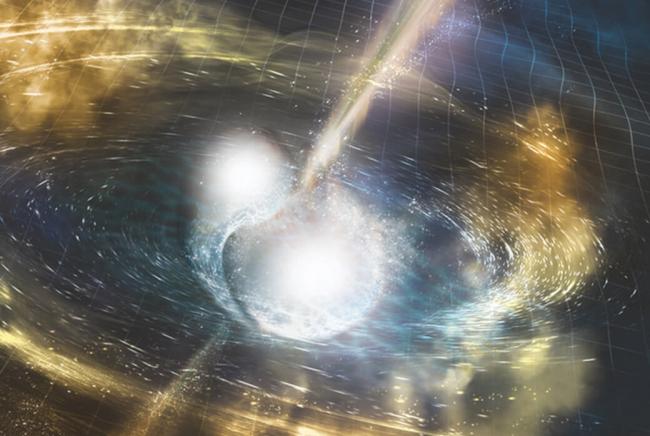
This artist’s illustration depicts two merging neutron stars and the gravitational waves they emit. As the LIGO and Virgo gravitational wave observatories have confirmed, collisions of black holes and neutron stars emit enough gravitational waves to be seen billions of light-years away.
- Why do we need an extremely large telescope like the Giant Magellan Telescope?
- What happens to space time when cosmic objects collide?
- Black Holes
- Gravitational Lensing
- Gravitational Waves
- Very Long Baseline Interferometry
- High Energy Astrophysics
- Optical and Infrared Astronomy
- Theoretical Astrophysics
Related News
Giant magellan telescope mount fabrication begins, m87* one year later: proof of a persistent black hole shadow, unveiling black hole spins using polarized radio glasses, the giant magellan telescope’s final mirror fabrication begins, new horizons in physics breakthrough prize awarded to cfa astrophysicist, cfa selects contractor for next generation event horizon telescope antennas, sheperd doeleman awarded the 2023 georges lemaître international prize, 'the dawn of a new era in astronomy', astronomers reveal first image of the black hole at the heart of our galaxy, bringing black holes to light, physics of the primordial universe, sensing the dynamic universe, castles survey, telescopes and instruments, event horizon telescope (eht), giant magellan telescope, the greenland telescope.
Subscribe or renew today
Every print subscription comes with full digital access
Science News
Einstein’s genius changed science’s perception of gravity.
General relativity has grown more important than it was in Einstein's day

GETTING A GRIP ON GRAVITY Einstein's general theory of relativity explains gravity as a distortion of space (or more precisely, spacetime) caused by the presence of matter or energy. A massive object generates a gravitational field by warping the geometry of the surrounding spacetime.
Nicolle Rager Fuller
Share this:
By Tom Siegfried
October 4, 2015 at 5:30 am
Albert Einstein opened humankind’s eyes to the universe.
Before Einstein, space seemed featureless and changeless, as Isaac Newton had defined it two centuries earlier. And time, Newton declared, flowed at its own pace, oblivious to the clocks that measured it. But Einstein looked at space and time and saw a single dynamic stage — spacetime — on which matter and energy strutted, generating sound and fury, signifying gravity.
Newton’s law of gravity had united the earthly physics of falling apples with the cosmic dances of planets and stars. But he couldn’t explain how, and he famously refused to try. It took an Einstein to figure out gravity’s true modus operandi. Gravity, Einstein showed, did not just make what goes up always come down. Gravity made the universe go ’round.
Gravity’s secrets succumbed to Einstein’s general theory of relativity, unveiled in a series of papers submitted a century ago this November to the Prussian Academy in Berlin. A decade earlier, his special theory of relativity had merged matter with energy while implying the unity of space and time (soon to be christened as spacetime). After years of struggle, Einstein succeeded in showing that matter and spacetime mutually interact to mimic Newton’s naïve idea that masses attract each other. Gravity, said Einstein, actually moved matter along the curving pathways embodied in spacetime — paths imprinted by mass and energy themselves. As expressed decades later by the physicist John Archibald Wheeler, mass grips spacetime, telling it how to curve, and spacetime grips mass, telling it how to move.
Einstein’s theory explained a famous observation that Newtonian gravity could not: a subtlety in the orbit of the planet Mercury. And his equations implied further slight deviations from Newtonian calculations. Over the last century, general relativity’s predictions have been repeatedly verified by modern precision measurements. To physicists today, general relativity and gravity are essentially synonyms.
Timeline: Einstein and relativity
But general relativity is about more than just understanding gravity. It’s about explaining the totality of existence. General relativity inspired a new vision of the entire fabric of the cosmos. From general relativity flowed the realization that the universe is expanding, that it contains spacetime bottomless pits called black holes, that it is traversed by ripples in space triggered by cataclysmic collisions.
“The implications for the further reaches of the universe were more surprising than even Einstein ever realized,” physicist Stephen Hawking has written.
General relativity explains how the universe can obey physical laws that apply to any form of motion. It’s at the heart of identifying and investigating crucial questions about space and time, existence and reality. And its implications are not limited to esoteric concerns on cosmic scales — it has its down-to-Earth impacts as well. Without general relativity, for instance, GPS devices would be worthless. Satellite signals designed to keep your car on the right road would be off by miles if not corrected for the effects predicted by Einstein’s math.
Gravitation revolution
On his road to general relativity, Einstein himself took many wrong turns. From 1907 to 1914 he struggled with what the physicist Abraham Pais called “one of the hardest problems of the century” — explaining gravity in a way that permits the laws of nature to be the same for all observers, no matter how they are moving. Einstein had to learn new math and jettison common prejudices, such as the universal belief that Euclidean geometry described reality accurately. He struggled with distractions, both in his personal life and in physics problems posed by quantum theory. And he found that nature stubbornly refused to cooperate. By 1914 he had essentially given up, believing that a partially successful attempt — a sort of general relativity lite — was the best that nature would allow.
But then somehow Einstein’s brain rebooted. His theory began to solidify, and he swiftly composed four papers, one a week, during November 1915. By the last paper he had finally found the decisive equation that launched his gravitation revolution.
Four years later, general relativity made Einstein himself a celebrity. If gravity curves space, he had realized early on, a light beam passing near a massive object (say, the sun) would be deflected from its course. That deflection would shift the apparent position of the source of the light (say, a distant star). During a solar eclipse, such a shift could be photographed and measured. Such measurements, made during eclipse expeditions in 1919, confirmed Einstein’s calculation. Even without Twitter to spread the word, Einstein’s triumph sparked a media sensation.
“Lights all askew in the heavens, men of science more or less agog,” proclaimed one of the most famous newspaper headlines in science history, in the Nov. 10 New York Times . And from the Times of London on November 7: “Revolution in Science, New Theory of the Universe, Newtonian Ideas Overthrown.”
Einstein became a legend, his name forevermore synonymous with genius.

As it turned out, some bending of light would have been expected even with Newtonian gravity, as Johann von Soldner had calculated (unknown to Einstein) more than a century earlier. But Einstein predicted precisely twice as much bending as von Soldner had. And although the earliest measurements were crude, they were much closer to Einstein’s predictions than Newton’s. In subsequent eclipses, Einstein’s calculation has been repeatedly confirmed. Gravity deflects light just as general relativity requires.
General relativity’s light-bending effect proved valuable for much more than affirming Einstein’s theory. By bending light, masses act like a lens; such “gravitational lensing” alters the apparent position of a distant object, creating multiple images of it, or (if the images overlap) appearing to brighten it. Such effects can be used to probe matter’s distribution in space or detect the presence of unseen masses.
“Since the discovery of the first gravitational lens, the phenomenon has been exploited to map the distribution of mass around galaxies and clusters, and to search for dark matter, dark energy, compact objects, and extrasolar planets,” physicist Clifford Will notes in a recent paper .
Gravitational lensing was first observed in 1979, but Einstein had suspected its possibility in 1912, even before his theory had been completed. Only in 1936 did he publish a paper about it, Will notes, “primarily, it seems, to get a Czech electrical engineer named Rudi Mandl to stop pestering him about it.” Will, of the University of Florida in Gainesville, does not mention that Mandl had first approached Science News Letter ( Science News ’ predecessor) with the gravitational lens idea; the magazine paid his expenses to visit Einstein, who then agreed to do the calculation that Mandl had suggested ( SNL: 12/19/36, p. 388 ). Einstein’s paper, published in Science, suggested that the effect would never be noticed. But modern astronomical explorations proved the contrary.
Einstein was also ambivalent about other consequences of general relativity. In 1916, for instance, he raised the possibility of gravitational radiation — waves rippling through spacetime after a massive body abruptly changes its motion, as when colliding with another mass. Such waves should exist, Einstein reasoned, because general relativity required gravity’s influence to propagate at the speed of light (whereas Newton’s gravity transmitted itself instantaneously). But later Einstein changed his mind. In 1936, he and Nathan Rosen submitted a paper arguing that such waves did not exist after all. But their paper contained a mistake. Today, gravitational waves’ reality has been established convincingly by indirect methods, and experiments to detect them directly are under way (see “Magnifying the Cosmos”).
Astronomers have already detected another offspring of general relativity, black holes, throughout the cosmos. But Einstein didn’t believe they would exist, either.
Explaining Mercury’s odd orbit

Black holes’ existence had been foreshadowed only weeks after Einstein presented his general relativity papers to the Prussian Academy. Karl Schwarzschild, a German astronomer serving in World War I on the Russian front, worked out solutions to Einstein’s complicated equations for the spacetime geometry around a massive sphere. It was the first mathematical step toward describing black holes in space. But Schwarzschild didn’t pursue the topic; he died a few months later from a skin disease. Not until the late 1960s did black holes emerge as general relativity’s most prominent advertisement, stimulating both science and the popular imagination. They became the most glamorous product of a theory conceived by science’s best imaginer.
Falling freely
Einstein’s imagination gave birth to general relativity’s core idea as he gazed out his office window while he was supposed to be evaluating patents. “All of a sudden I was struck by a thought,” Einstein later said. “If a person falls freely, he will certainly not feel his own weight.”
It was 1907, two years after his special theory of relativity had rewritten textbook notions about time and motion.
Special relativity showed that the laws of nature don’t depend on how you are moving, as long as it’s uniform motion — constant speed in a straight line. But in real life, objects and people move in all sorts of nonuniform ways. (Let the air out of a balloon, for instance.) Even some “simple” motions, like the rotation of a sphere or orbit of a planet, are nonuniform, as they constantly change direction and are therefore accelerating. Einstein wanted to extend relativity to all forms of accelerated motions. But he didn’t know how.
Then his happy thought in the patent office raised hope. A person falling freely accelerates toward the ground because of gravity but feels no force (until impact). Therefore, Einstein realized, gravity and acceleration are two sides of a coin. The upward thrust of an accelerating rocket ship pins the occupants to the floor just as the gravitational pull of the Earth keeps your feet on the ground. This acceleration-gravity equivalence explained a curious Newtonian coincidence: A body’s mass (its inertial resistance to changes in motion) is equal to its weight (or gravitational mass), its response to gravity. Einstein built special relativity on the principle that light’s velocity was constant; he suspected that general relativity could be built on the principle that inertial and gravitational mass are equivalent. If he succeeded, it would mean that nature’s laws could be the same for all forms of motion.
At first progress was slow. Then a key clue emerged in 1908, when the mathematician Hermann Minkowski showed how special relativity required the merger of space with time ( SN: 9/13/08, p. 26 ). In special relativity, measures of space or time differ for different observers. But Minkowski showed that space and time combined — spacetime — yielded a mathematical description of events that all observers could agree on. Any event’s location could be specified by a set of space and time coordinates.
Bending light
One of the first predictions of general relativity to be tested involved the bending of light. Because a massive body, such as a star, warps spacetime around it, a light beam passing nearby should be deflected from a straight-line path. From Earth, light from a distant star passing near the sun would be bent in such a way as to alter the apparent position of the distant star. In 1919, astronomers photographed stars near the sun during a solar eclipse. Comparing the positions of the stars in those photos with nighttime photos taken earlier showed that the stars’ positions did appear to shift by about the amount Einstein predicted. Actually, calculations based on Newton’s gravity also predicted light bending, but only by half as much as Einstein calculated. General relativity requires twice as much bending because the light ray is bent while passing through space already curved to begin with.

Establishing such coordinates requires a frame of reference, or origin point. Different observers will choose different origins. So if nature’s laws are the same for everybody, then any one person’s set of coordinates should in some sense be equivalent to anybody else’s. Einstein’s quest, then, became an effort to find the formula for transforming any one coordinate system into any other, while maintaining the equivalence between gravity and acceleration.
By 1912, Einstein realized that his goal would require abandoning Euclidean geometry. Real space, he realized, could not conform to the idealized lines and angles of the textbooks. Gravity distorted the coordinates, just as a grid of straight lines on a rubber sheet would curve if you placed a heavy cannonball on it.
But Einstein did not possess the mathematical skills to cope with non-Euclidean geometry. Fortunately, his college friend Marcel Grossmann, an accomplished mathematician, was eager to help. Familiar with 19th century–mathematician Bernhard Riemann’s work on the math for describing curved surfaces, Grossmann helped Einstein produce the outline ( Entwurf in German) of a new gravity theory. It had one drawback, though — it worked for some coordinate systems, but not all possible systems. Einstein was dismayed, writing to the physicist Hendrik Lorentz in August 1913 that “there are still such major snags in the thing that my confidence in the admissibility of the theory is still shaky.” If acceleration is equivalent to a gravitational field, Einstein noted, every kind of acceleration should be describable by the equations for gravity. If not, “the theory refutes its own starting point; then it has no foundation whatsoever.”
Two days later, Einstein seemed much happier, writing to Lorentz that the Entwurf theory’s deficiency had been solved. In November, Einstein described that solution in a letter to physicist Paul Ehrenfest, asserting that equations describing all accelerations simply couldn’t exist; some coordinate systems get special status in order to preserve the law of energy-momentum conservation. That made his original goal impossible. But Einstein seemed satisfied that he had done the best that nature would permit.
“Can there be anything more beautiful than this, that the necessary specialization follows from the conservation laws?” Einstein asked Ehrenfest. It turned out that there actually was something more beautiful. But to find it, Einstein had to move to Berlin.
Breakthrough in Berlin
Einstein’s life until then had been peripatetic. Born in Ulm, Germany, in 1879, he moved when an infant to Munich and then as a teenager to Milan, Italy, having dropped out of high school. He went back to school in Switzerland, eventually graduating from college in Zurich. Failing to find an academic job, he joined the Swiss patent office in 1902, and the next year married Mileva Marić.
During his years at the patent office, Einstein produced an explosive output of papers poking holes in traditional physics, including reports on his special theory of relativity and Nobel Prize–winning work on quantum physics. Eventually those papers led to sufficient recognition in the physics world to get a faculty appointment in Prague. But at the first chance he returned to Zurich, where Grossmann now taught math. There Einstein and Grossmann developed the Entwurf theory. And then Berlin, the pinnacle of German (and world) physics, called. Prominent physicists at the university there made Einstein an offer he couldn’t refuse: no teaching responsibilities. As he wrote to Lorentz: “I could not resist the temptation to accept a position in which I am relieved of all responsibilities so that I can give myself over completely to rumination.”
Story continues below box
The Einstein field equations
Because mass and energy distort the shape of spacetime, the Euclidean geometry of standard textbooks can’t accurately describe it. Einstein’s general relativity uses more complicated math built on the non-Euclidean geometry devised in the 19th century by Bernhard Riemann. With help from his friend Marcel Grossmann, Einstein adopted further advances by the mathematicians Gregorio Ricci-Curbastro, Tullio Levi-Civita and Elwin Christoffel to describe spacetime geometry in terms of mathematical expressions called tensors. Tensors are a lot like vectors — quantities, such as velocity, composed of two components (in velocity’s case, speed and direction). Tensors are similar but can encompass more than just two components. Einstein used tensors to develop his equation describing the gravitational field, known as the Einstein field equation.
The Einstein Field Equation
Gμν = 8πtμν.
On the left side of the equation is a tensor describing the geometry of spacetime — the gravitational field. On the right is the tensor describing the matter and energy density — the source of the gravitational field. The equation shows that spacetime geometry equals mass-energy density when adjusted with the proper units and numerical constants. (Actually, the equation stands for a set of multiple equations owing to the complexity of tensors. So experts usually speak of the Einstein field equations, plural.)
The Einstein Field Equation with Lambda
Gμν + λgμν = 8πtμν.
When he applied this equation to the entire universe, Einstein found that the universe would be unstable, easily disturbed into a state in which spacetime would be either expanding or collapsing. So he added a term that came to be called the cosmological constant, symbolized by the Greek letter lambda. It represents a constant amount of energy density throughout space that would supposedly keep the universe stable and changeless. Later, evidence that the universe was indeed expanding led Einstein to renounce lambda. But it has been revived by modern cosmologists to explain the apparent increase in the universe’s rate of expansion that was discovered in the late 1990s.
Throughout all these moves, Einstein’s personal life deteriorated. Growing apart from Mileva, he pursued a relationship with his cousin Elsa. And Mileva did not relish the idea of life in Berlin. In July 1914 she took their two sons back to Zurich, and Einstein was left in Berlin free to ruminate about general relativity to his heart’s content. During the following year his freedom nourished a fertile few months in which he saw a new path to success. In mid-1915 he saw that there was a way to make relativity truly general. Rather than imposing energy-momentum conservation on the equations, he worked on devising equations that would impose the conservation law on the universe.
Einstein now applied the full force of all the mathematical wizardry he had mastered in the preceding years, adopting embellishments of Riemann’s math called tensors. By November 1915, Einstein sensed victory. On November 4, he submitted a paper on general relativity to the Prussian Academy, following up with an addendum November 11. The next week, he presented yet another new paper, this time in a lecture to the academy, showing how general relativity’s spacetime curvature solved the outstanding gravitational problem in all of physics, the anomaly in the orbit of Mercury. During the following week, he finally found the correct form for the equations describing the gravitational field, presenting his result on November 25. Einstein’s quest was completed. General relativity worked.
Universal implications
Einstein quickly realized (or had known all along) that his new theory of gravity was really a theory of the cosmos. In 1917, he wrote a famous paper applying general relativity to the universe as a whole. Today that paper stands as the foundation for modern cosmology. But at the time, Einstein was troubled — his equations implied an unstable universe, either growing or collapsing. In those days, the universe was supposed to be eternal, everlasting and changeless. So Einstein altered his equation, adding a factor called the cosmological constant, representing a constant energy density in space that kept the universe static.
Others didn’t buy it. Alexander Friedmann, a Russian meteorologist-mathematician, developed a description of an expanding or contracting universe from Einstein’s original equations. Einstein first thought Friedmann to be in error, but then relented, although still viewing the “expanding universe” as of mathematical interest only. But a few years later, when Edwin Hubble’s analysis of light from distant galaxies confirmed the universe’s expansion, Einstein gave in. Despite his own reluctance to accept it, Einstein’s general relativity math did in fact imply what Wheeler later called the “most dramatic prediction that science has ever made” — the expansion of the universe.
Today, Einstein’s cosmological constant has been revived. Rather than preventing the universe from collapse, the vacuum energy it describes can explain why the universe now expands at an accelerating pace. General relativity, cosmological constant and all, today forms the core science for analyzing the history of the universe and forecasting its future.
But apart from its use in cosmology, general relativity was not widely applied to scientific problems in its first four decades. For the most part, general relativity languished in departments of mathematics, rarely studied in physics.
Gravitational collapse

At the time, nobody paid any attention. But in the 1960s, newfound astrophysical anomalies suggested that gravitational collapse was at work in the cosmos, and Oppenheimer and Snyder’s idea was revived as what came to be known as black holes. Famous for swallowing anything they encounter and allowing nothing to escape, black holes are probably the most bizarre astrophysical consequences of general relativity. Small black holes have been detected throughout space and supermassive black holes reside in the cores of most galaxies.
More recently black holes (schematic of one shown) have been used as thought-experiment laboratories for investigating several outstanding mysteries about the nature of space and time.
“General relativity was essentially a dead subject for a long time,” says the prominent relativity theorist Wolfgang Rindler of the University of Texas at Dallas. “People considered it a dormant science.”
Only after Einstein died in 1955 did general relativity come to life. About that time Wheeler, at Princeton University, began a program to explore its implications and train students to pursue them. By the early 1960s, new astronomical phenomena demanded explanations that Newtonian physics could not provide, and general relativity was poised for its renaissance. In the decades that followed, general relativity proved crucial for describing all sorts of celestial phenomena. At the same time, physicists devised ever more precise tests of its predictions, and Einstein passed them all. As Will has noted, “It is remarkable that this theory, born 100 years ago out of almost pure thought, has managed to survive every test.”
Even harder than finding the equations for general relativity is explaining how, out of almost pure thought, Einstein did it. Science historian Gerald Holton once remarked, while describing Einstein in the context of defining scientific genius, that “there is a mutual mapping of the mind and lifestyle of this scientist, and of the laws of nature.” Einstein himself ascribed his success to discovering the deep relationship between mathematics and the physical world.
In creating general relativity, Einstein’s path was forked; he had to envision physical processes governing matter, space and time, while at the same time formulating abstract mathematical expressions corresponding to that reality. As a student, Einstein testified, he neglected mathematics. His intuition was not strong enough to guide him to the most profound of math’s many subfields. But in the physical realm of natural phenomena, “I soon learned to scent out that which was able to lead to fundamentals and to turn aside from … the multitude of things which clutter up the mind and divert it from the essential.” At first he didn’t realize that “a more profound knowledge of the basic principles of physics is tied up with the most intricate mathematical methods.” He learned that from his pursuit of general relativity.
In the end, Einstein’s mind produced a mathematical theory so rich that it entailed unsuspected cosmic novelties. Fantastic physical phenomena were first discovered not through the lenses of telescopes, but within the squiggles Einstein had scratched out on paper to make the world make sense — to him. And now physical nature makes sense to modern science only because of Einstein’s insights.
“Einstein’s ideas,” his friend the physicist Max Born wrote over half a century ago, “have given the physical sciences the impetus which has liberated them from outdated philosophical doctrine, and made them one of the decisive factors in the modern world of man.”
Gravity’s pull on time

General relativity and GPS

This article appears in the October 17, 2015, Science News with the headline, “Getting a grip on gravity: Einstein’s genius reconstructed science’s perception of the cosmos.”
More Stories from Science News on Astronomy

A cosmic census triples the known number of black holes in dwarf galaxies

A star winked out of sight. Could it be a ‘failed supernova’?

A zombie star’s spiky filaments shed light on a 12th century supernova

Using AI, historians track how astronomy ideas spread in the 16th century

Runaway stars could influence the cosmos far past their home galaxies

50 years ago, satellites threatened astronomers’ view of the cosmos

Barnard’s star has at least one planet orbiting it after all


Betelgeuse has a tiny companion star hidden in plain sight
Subscribers, enter your e-mail address for full access to the Science News archives and digital editions.
Not a subscriber? Become one now .

The Happy Scientist
Error message, is gravity a theory or a law.
I frequently get emails wanting to know whether gravity is a law or a theory. That question brings up so many more questions that I thought it would be fun to explore.
To try this, you will need:
- an object to drop.
OK, pick an object that will not break, dent the floor, cause a mess, or get either of us in trouble. Hold it out in front of you and release it. What happens? It falls, unless you picked a helium balloon. In that case, gravity causes it to float upwards, by pulling downwards with more force on the air around the balloon). The gravitational attraction between the Earth and the object pulls it towards the ground. But, when we do this experiment, should we be talking about the Law of Gravity or the Theory of Gravity?
Actually, we should be talking about both. To understand why, we need to understand the scientific meaning of the words "law" and "theory."
In the language of science, the word "law" describes an analytic statement. It gives us a formula that tells us what things will do. For example, Newton's Law of Universal Gravitation tells us:
"Every point mass attracts every single point mass by a force pointing along the line intersecting both points. The force is directly proportional to the product of the two masses and inversely proportional to the square of the distance between the point masses."
So if we know the mass of two objects, and the distance between the center of mass of the two objects, we can calculate the gravitational pull between the Earth and the object you dropped, between the Sun and Mars, or between me and a bowl of ice cream.
We can use Newton's Law of Universal Gravitation to calculate how strong the gravitational pull is between the Earth and the object you dropped, which would let us calculate its acceleration as it falls, how long it will take to hit the ground, how fast it would be going at impact, how much energy it will take to pick it up again, etc.
While the law lets us calculate quite a bit about what happens, notice that it does not tell us anything about WHY it happens. That is what theories are for. In the language of science, a theory is an explanation of why and how things happen. For gravity, we use Einstein's Theory of General Relativity to explain why things fall.
A theory starts as one or more hypotheses, untested ideas about why something happens. For example, I might propose a hypothesis that the object that you released fell because it was pulled by the Earth's magnetic field. Once we started testing, it would not take long to find out that my hypothesis was not supported by the evidence. Non-magnetic objects fall at the same rate as magnetic objects. Because it was not supported by the evidence, my hypothesis does not gain the status of being a theory. To become a scientific theory, an idea must be thoroughly tested, and must be an accurate and predictive description of the natural world.
While laws rarely change, theories change frequently as new evidence is discovered. Instead of being discarded because of new evidence, theories are often revised to include the new evidence in their explanation. The Theory of General Relativity has adapted as new technologies and new evidence have expanded our view of the universe.
So when we are scientifically discussing gravity, we can talk about the law of gravity that describes the attraction between two objects, and we can also talk about the theory of gravity that describes why the objects attract each other.
When Does a Theory Become a Law?
Please log in.
Search form
Search by topic, search better.
- Life Science
- Earth Science
- Chemical Science
- Space Science
- Physical Science
- Process of Science
Related Posts

What is Science?
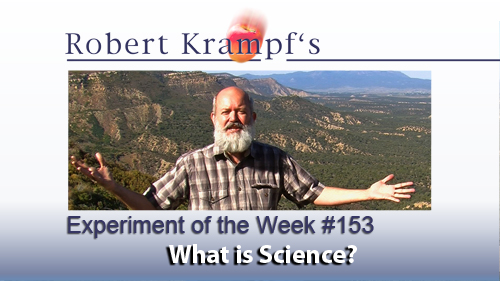
My Position on Science and Religion
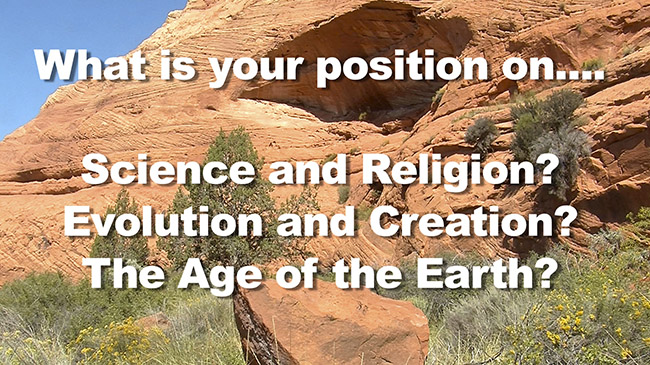
- The Magazine
- Stay Curious
- The Sciences
- Environment
- Planet Earth
How to Understand Einstein's Theory of Gravity
Einstein's general relativity may be complicated, but it's our best way of understanding the universe..
An astronaut wakes up in a spaceship, with no memory of how she got there. Sitting alone in a chair, she wonders: “Where in the universe am I?”
The ship has no windows. Its instruments are dead. The only clue is the push of the chair against her body. Phew, there’s gravity, she thinks. Her vessel must still be on Earth.
But then a second possibility occurs to her. The ship could be accelerating through space, pressing her into the seat like a race car picking up speed. From inside the vessel, there is — terrifyingly — no way to tell.
This spacefarer’s dilemma would have been familiar to Albert Einstein. His 1915 general theory of relativity built on the notion that gravity and acceleration are not just easily confused, but are one and the same. This equivalence, “the happiest thought” of Einstein’s life, was his starting point for redefining gravity.
General relativity grew out of Einstein’s theory of special relativity, which describes how the speed of light (in a vacuum) can always be constant.
According to relativity, anything that can happen inside of a box picking up speed — i.e., accelerating — also happens in the presence of gravity. Imagine, for example, a horizontal laser inside an elevator that’s accelerating upward. As the light travels sideways, the elevator rises, causing the beam to strike a spot on the wall slightly lower than where it started. If the elevator accelerates quickly enough, the beam visibly bends toward the floor.
Einstein showed the same thing happens to a beam inside a stationary elevator within a powerful gravitational field; the gravity bends the light. Similarly, he expected a beam of starlight should bend when passing through the sun’s gravity. This prediction proved correct when the stars moved during the 1919 solar eclipse.
Relativity describes why a clock on a satellite ticks a few dozen microseconds faster than a clock on Earth; without accounting for that discrepancy, GPS technologies wouldn’t work.
To link acceleration and gravity in this way, Einstein overthrew one of his own heroes: Isaac Newton. You may have learned that Newton described gravity as a force, an invisible rubber band that pulls together objects with mass. Newton’s math did a good job at predicting how everything from projectiles to planets moved — but it kept gravity separate from acceleration.
Einstein argued that gravity isn’t a force at all. He described it as a curvature of time and space caused by mass and energy. Confused? The German physicist was, too, and he struggled with the theory for nearly a decade. He got help from mathematician Marcel Grossmann, an old friend who shared his notes when a young Einstein skipped class.
Their math, laid down in 10 equations, explained how gravity could move around objects via a warped reality, accelerating without ever feeling any mysterious Newtonian forces.
The Relative Basics
The main takeaways behind Einstein’s general theory of relativity:
1. Time and space are neither flat nor fixed; they are curved and distorted by mass and energy.
2. Gravity is not a force, but rather a distortion of time and space.
3. The effects of gravity are indistinguishable from the effects of acceleration, over a small space.
Einstein’s Peculiar Predictions
Relativity makes numerous bizarre predictions, many of them experimentally verified. They only seem bizarre because we don’t notice them in our daily lives — we live, for the most part, in Newton’s reality. But beyond that lies Einstein’s universe, where gravity bends space and time to its will. Here are some of the theory’s strangest side effects:
Gravity literally slows down time. Waves of light emitted by stars stretch out because of this time bending, and objects closer to a massive object age more slowly. Super-precise clocks, which tick according to the vibrations of atoms, have verified that gravity alters time’s flow.
Satellites have shown that rotating celestial bodies twirl the fabric of the cosmos around themselves, like honey twisted by a spoon, affecting the motion of gyroscopes.
One prediction solved a long-standing dilemma, a weird wobble in Mercury’s orbit that Newton’s math couldn’t account for. (Astronomers had initially blamed a hidden planet called Vulcan.) Relativity explained the wonky orbit in terms of the warping of space by the sun’s powerful gravity.
Tiny ripples in reality, caused by colliding black holes, have tripped sensors in highly sensitive instruments buried underground on Earth.
This story originally appeared in print as "It's All Relative."
Already a subscriber?
Register or Log In
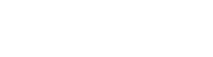
Keep reading for as low as $1.99!
Sign up for our weekly science updates.
Save up to 40% off the cover price when you subscribe to Discover magazine.

What is the theory of general relativity? Understanding Einstein's space-time revolution
Here we explore what the theory of general relativity is and how it affects space-time.
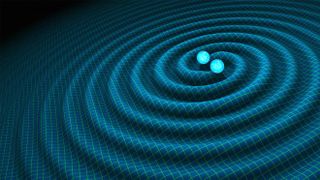
- How it works
General relativity FAQs answered by an expert
General relativity and gravitational lensing.
- Mercury's orbit
- Frame-dragging
Gravitational redshift
Gravitational waves.
- Neutron stars
Additional resources
General relativity is physicist Albert Einstein's understanding of how gravity affects the fabric of space-time.
The theory, which Einstein published in 1915, expanded the theory of special relativity that he had published 10 years earlier. Special relativity argued that space and time are inextricably connected, but that theory didn't acknowledge the existence of gravity .
Einstein spent the decade between the two publications determining that particularly massive objects warp the fabric of space-time , a distortion that manifests as gravity, according to NASA .
Related: The hunt for wormholes: How scientists look for space-time tunnels
- Albert Einstein: Before and After Relativity
- Fast-Spinning Star Tests Einstein's General Relativity Theory
How does general relativity work?
To understand general relativity, first, let's start with gravity, the force of attraction that two objects exert on one another. Sir Isaac Newton quantified gravity in the same text in which he formulated his three laws of motion, the " Principia ."
The gravitational force tugging between two bodies depends on how massive each one is and how far apart the two lie, according to NASA Glenn Research Center . Even as the center of the Earth is pulling you toward it (keeping you firmly lodged on the ground), your center of mass is pulling back at the Earth. But the more massive body barely feels the tug from you, while with your much smaller mass, you find yourself firmly rooted thanks to that same force. Yet Newton's laws assume that gravity is an innate force of an object that can act over a distance.
Albert Einstein, in his theory of special relativity , determined that the laws of physics are the same for all non-accelerating observers, and he showed that the speed of light within a vacuum is the same no matter the speed at which an observer travels, according to Wired .
As a result, he found that space and time were interwoven into a single continuum known as space-time. And events that occur at the same time for one observer could occur at different times for another.
Related: What would happen if the speed of light was much lower?
As he worked out the equations for his general theory of relativity, Einstein realized that massive objects caused a distortion in space-time. Imagine setting a large object in the center of a trampoline. The object would press down into the fabric, causing it to dimple. If you then attempt to roll a marble around the edge of the trampoline, the marble would spiral inward toward the body, pulled in much the same way that the gravity of a planet pulls at rocks in space.
In the decades since Einstein published his theories, scientists have observed countless of phenomena matching the predictions of relativity.
We asked Elena Giorgi, an assistant professor of mathematics at Columbia University a few commonly asked questions about general relativity.
Elena Giorgi is an assistant professor of mathematics at Columbia University in the group of Geometry & Analysis and Mathematical Physics. Giorgi's research interests are general relativity, partial differential equations and differential geometry.
What is general relativity?
General relativity is a physical theory about space and time and it has a beautiful mathematical description. According to general relativity, the spacetime is a 4-dimensional object that has to obey an equation, called the Einstein equation, which explains how the matter curves the spacetime.
What force is explained by general relativity?
General relativity explains gravity, and in this theory, it is not really a "force" anymore. The gravitational field comes out of the description of general relativity as a result of the curved spacetime.
When was the theory of general relativity established?
General Relativity was established in 1915 by Albert Einstein and the first solutions to the Einstein equation were found already in early 1916.
Is general relativity proven?
General relativity has passed all the experimental tests so far, but its applicability is expected to break down when [the] effects of quantum mechanics (the theory of the very small particles) should become dominant.
Light bends around a massive object, such as a black hole, causing it to act as a lens for the things that lie behind it. Astronomers routinely use this method to study stars and galaxies behind massive objects.
The Einstein Cross, a quasar in the Pegasus constellation , according to the European Space Agency (ESA), and is an excellent example of gravitational lensing. The quasar is seen as it was about 11 billion years ago; the galaxy that it sits behind is about 10 times closer to Earth. Because the two objects align so precisely, four images of the quasar appear around the galaxy because the intense gravity of the galaxy bends the light coming from the quasar.
Related: What is quantum gravity?
In cases like Einstein's cross, the different images of the gravitationally lensed object appear simultaneously, but that isn't always the case. Scientists have also managed to observe lensing examples where, because the light traveling around the lens takes different paths of different lengths, different images arrive at different times, as in the case of one particularly interesting supernova .
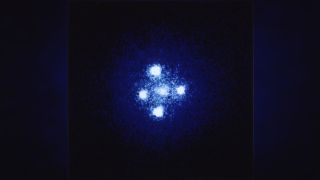
General relativity and changes in Mercury's orbit
The orbit of Mercury is shifting very gradually over time due to the curvature of space-time around the massive sun, according to NASA .
As the closest planet to the sun, Mercury’s perihelion (the point along its orbit that it’s closest to the sun) is predicted to follow a slightly different direction over time. Under Newton’s predictions, gravitational forces in the solar system should advance Mercury's precession ( change in its orbital orientation) is measured to be 5,600 arcseconds per century (1 arcsecond is equal to 1/3600 of a degree). However, there is a discrepancy of 43 arcseconds per century, something Einstein's theory of general relativity accounts for. Using Einstein’s theory of curved space-time, the precession of Mercury’s perihelion should advance slightly more than under the predictions of Newton, since planets don’t orbit the sun in a static elliptical orbit.
Sure enough, several research papers published since the mid 20th century have confirmed Einstein's calculations of Mercury’s perihelion precession to be accurate.
In a few billion years, this wobble could even cause the innermost planet to collide with the sun or a planet .
Frame-dragging of space-time around rotating bodies
The spin of a heavy object, such as Earth, should twist and distort the space-time around it. In 2004, NASA launched the Gravity Probe B (GP-B). The axes of the satellite's precisely calibrated gyroscopes drifted very slightly over time, according to NASA , a result that matched Einstein's theory.
"Imagine the Earth as if it were immersed in honey," Gravity Probe-B principal investigator Francis Everitt, of Stanford University, said in a NASA statement about the mission.
"As the planet rotates, the honey around it would swirl, and it's the same with space and time. GP-B confirmed two of the most profound predictions of Einstein's universe, having far-reaching implications across astrophysics research."
The electromagnetic radiation of an object is stretched out slightly inside a gravitational field . Think of the sound waves that emanate from a siren on an emergency vehicle; as the vehicle moves toward an observer, sound waves are compressed, but as it moves away, they are stretched out, or redshifted . Known as the Doppler Effect, the same phenomena occurs with waves of light at all frequencies.
In the 1960s, according to the American Physical Society , physicists Robert Pound and Glen Rebka shot gamma-rays first down, then up the side of a tower at Harvard University. Pound and Rebka found that the gamma-rays slightly changed frequency due to distortions caused by gravity.
Einstein predicted that violent events, such as the collision of two black holes, create ripples in space-time known as gravitational waves . And in 2016, the Laser Interferometer Gravitational Wave Observatory ( LIGO ) announced that it had detected such a signal for the first time.
That detection came on Sept. 14, 2015 . LIGO, made up of twin facilities in Louisiana and Washington, had recently been upgraded, and were in the process of being calibrated before they went online. The first detection was so large that, according to then-LIGO spokesperson Gabriela Gonzalez, it took the team several months of analysis to convince themselves that it was a real signal and not a glitch.
Related: Phantom energy and dark gravity: Explaining the dark side of the universe
"We were very lucky on the first detection that it was so obvious," she said during the 228 American Astronomical Society meeting in June 2016.
Since then, scientists have begun quickly catching gravitational waves. All told LIGO and its European counterpart Virgo have detected over 100 gravitational-wave events, according to the Gravitational Wave Open Science Center .
Those collisions have included unusual events like a collision with an object that scientists can't definitively identify as a black hole or neutron star, merging neutron stars accompanied by a bright explosion, mismatched black holes colliding and more.
Observing neutron stars
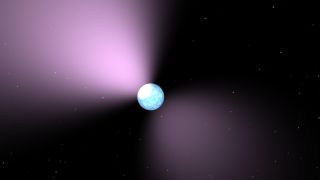
In 2021 research published in the journal Physical Review X , challenged several of Einstein's predictions by observing a double-pulsar system around 2,400 light-years from Earth. Each of the seven predictions of general relativity was confirmed by the study.
Pulsars are a type of neutron star that appears to pulse due to beams of electromagnetic radiation and that are emitting from their magnetic poles.
The pulsar test subjects spin very fast - around 44 times a second - and are 30% more massive than the sun but are only 15 miles (around 24 kilometers) in diameter, making them incredibly dense. This means that their gravitational pull is immense, for example, on the surface of a neutron star gravity is around 1 billion times stronger than its pull on Earth. This makes neutron stars a great test subject to challenge predictions in Einstein's theories, such as the ability of gravity to bend light.
"We follow the propagation of radio photons emitted from a cosmic lighthouse, a pulsar, and track their movements in the strong gravitational field of a companion pulsar," Professor Ingrid Stairs from the University of British Columbia at Vancouver said in a statement.
"We see for the first time how the light is not only delayed due to a strong curvature of spacetime around the companion but also that the light is deflected by a small angle of 0.04 degrees that we can detect. Never before has such an experiment been conducted at such a high spacetime curvature" Stairs adds.
Read more about general relativity in the book Relativity: The Special and the General Theory - 100th Anniversary Edition . Explore the Nature of Space and Time (Isaac Newton Institute Series of Lectures, 3) and The Physics Book: Big Ideas Simply Explained .
Join our Space Forums to keep talking space on the latest missions, night sky and more! And if you have a news tip, correction or comment, let us know at: [email protected].
Get the Space.com Newsletter
Breaking space news, the latest updates on rocket launches, skywatching events and more!
Nola Taylor Tillman is a contributing writer for Space.com. She loves all things space and astronomy-related, and enjoys the opportunity to learn more. She has a Bachelor’s degree in English and Astrophysics from Agnes Scott college and served as an intern at Sky & Telescope magazine. In her free time, she homeschools her four children. Follow her on Twitter at @NolaTRedd
Where did the universe's magnetic fields come from?
Aurora activity is just getting started. Here's why the best northern lights are yet to come.
'Silo' Season 2 Episode 1: What happened to Silo 17's tragic rebel uprising?
Most Popular
- 2 SpaceX launching 1st mission for Indian Space Research Organisation today
- 3 What to expect during SpaceX's 6th Starship test flight on Nov. 19
- 4 SpaceX launches 20 Starlink satellites from California (photos)
- 5 SpaceX launches telecom satellite from Florida in gorgeous sunset liftoff (video, photos)
- Science, Tech, Math ›
- Physics Laws, Concepts, and Principles ›
The History of Gravity
- Physics Laws, Concepts, and Principles
- Quantum Physics
- Important Physicists
- Thermodynamics
- Cosmology & Astrophysics
- Weather & Climate
:max_bytes(150000):strip_icc():format(webp)/AZJFaceShot-56a72b155f9b58b7d0e783fa.jpg)
- M.S., Mathematics Education, Indiana University
- B.A., Physics, Wabash College
One of the most pervasive behaviors that we experience, it's no wonder that even the earliest scientists tried to understand why objects fall toward the ground. The Greek philosopher Aristotle gave one of the earliest and most comprehensive attempts at a scientific explanation of this behavior by putting forth the idea that objects moved toward their "natural place."
This natural place for the element of Earth was in the center of the Earth (which was, of course, the center of the universe in Aristotle's geocentric model of the universe). Surrounding the Earth was a concentric sphere that was the natural realm of water, surrounded by the natural realm of air, and then the natural realm of fire above that. Thus, Earth sinks in water, water sinks in the air, and flames rise above air. Everything gravitates toward its natural place in Aristotle's model, and it comes across as fairly consistent with our intuitive understanding and basic observations about how the world works.
Aristotle further believed that objects fall at a speed that is proportional to their weight. In other words, if you took a wooden object and a metal object of the same size and dropped them both, the heavier metal object would fall at a proportionally faster speed.
Galileo and Motion
Aristotle's philosophy about motion toward a substance's natural place held sway for about 2,000 years, until the time of Galileo Galilei . Galileo conducted experiments rolling objects of different weights down inclined planes (not dropping them off the Tower of Pisa, despite the popular apocryphal stories to this effect), and found that they fell with the same acceleration rate regardless of their weight.
In addition to the empirical evidence, Galileo also constructed a theoretical thought experiment to support this conclusion. Here is how the modern philosopher describes Galileo's approach in his 2013 book Intuition Pumps and Other Tools for Thinking :
"Some thought experiments are analyzable as rigorous arguments, often of the form reductio ad absurdum , in which one takes one's opponents' premises and derives a formal contradiction (an absurd result), showing that they can't all be right. One of my favorites is the proof attributed to Galileo that heavy things don't fall faster than lighter things (when friction is negligible). If they did, he argued, then since heavy stone A would fall faster than light stone B, if we tied B to A, stone B would act as a drag, slowing A down. But A tied to B is heavier than A alone, so the two together should also fall faster than A by itself. We have concluded that tying B to A would make something that fell both faster and slower than A by itself, which is a contradiction."
Newton Introduces Gravity
The major contribution developed by Sir Isaac Newton was to recognize that this falling motion observed on Earth was the same behavior of motion that the Moon and other objects experience, which holds them in place within relation to each other. (This insight from Newton was built upon the work of Galileo, but also by embracing the heliocentric model and Copernican principle , which had been developed by Nicholas Copernicus prior to Galileo's work.)
Newton's development of the law of universal gravitation, more often called the law of gravity , brought these two concepts together in the form of a mathematical formula that seemed to apply to determine the force of attraction between any two objects with mass. Together with Newton's laws of motion , it created a formal system of gravity and motion that would guide scientific understanding unchallenged for over two centuries.
Einstein Redefines Gravity
The next major step in our understanding of gravity comes from Albert Einstein , in the form of his general theory of relativity , which describes the relationship between matter and motion through the basic explanation that objects with mass actually bend the very fabric of space and time (collectively called spacetime). This changes the path of objects in a way that is in accord with our understanding of gravity. Therefore, the current understanding of gravity is that it is a result of objects following the shortest path through spacetime, modified by the warping of nearby massive objects. In the majority of cases that we run into, this is in complete agreement with Newton's classical law of gravity. There are some cases which require the more refined understanding of general relativity to fit the data to the required level of precision.
The Search for Quantum Gravity
However, there are some cases where not even general relativity can quite give us meaningful results. Specifically, there are cases where general relativity is incompatible with the understanding of quantum physics .
One of the best known of these examples is along the boundary of a black hole , where the smooth fabric of spacetime is incompatible with the granularity of energy required by quantum physics. This was theoretically resolved by the physicist Stephen Hawking , in an explanation that predicted black holes radiate energy in the form of Hawking radiation .
What is needed, however, is a comprehensive theory of gravity that can fully incorporate quantum physics. Such a theory of quantum gravity would be needed in order to resolve these questions. Physicists have many candidates for such a theory, the most popular of which is string theory , but none which yield sufficient experimental evidence (or even sufficient experimental predictions) to be verified and broadly accepted as a correct description of physical reality.
Gravity-Related Mysteries
In addition to the need for a quantum theory of gravity, there are two experimentally-driven mysteries related to gravity that still need to be resolved. Scientists have found that for our current understanding of gravity to apply to the universe, there must be an unseen attractive force (called dark matter) that helps hold galaxies together and an unseen repulsive force (called dark energy ) that pushes distant galaxies apart at faster rates.
- Newton's Law of Gravity
- Einstein's Theory of Relativity
- What Is Buoyant Force? Origins, Principles, Formulas
- Two-Dimensional Kinematics or Motion in a Plane
- Introduction to Newton's Laws of Motion
- The Relationship Between Electricity and Magnetism
- History of the Michelson-Morley Experiment
- What Is Young's Modulus?
- What Is Magnetism? Definition, Examples, Facts
- What Is the Shear Modulus?
- How Does Static Electricity Work?
- What Is an Electric Field? Definition, Formula, Example
- The Science of Magnetic Field Lines
- One-Dimensional Kinematics: Motion Along a Straight Line
- What Is Natural Frequency?
- Doppler Effect in Light: Red & Blue Shift

IMAGES
VIDEO
COMMENTS
Our modern understanding of gravity comes from Albert Einstein's theory of general relativity, which stands as one of the best-tested theories in science. General relativity predicted many phenomena years before they were observed, including black holes, gravitational waves, gravitational lensing, the expansion of the universe, and the different rates clocks run in a gravitational field.
Newton's law of gravity had united the earthly physics of falling apples with the cosmic dances of planets and stars. But he couldn't explain how, and he famously refused to try. It took an ...
That is what theories are for. In the language of science, a theory is an explanation of why and how things happen. For gravity, we use Einstein's Theory of General Relativity to explain why things fall. A theory starts as one or more hypotheses, untested ideas about why something happens. For example, I might propose a hypothesis that the ...
Gravity - Force, Physics, Theory: The Newtonian theory of gravity is based on an assumed force acting between all pairs of bodies—i.e., an action at a distance. When a mass moves, the force acting on other masses had been considered to adjust instantaneously to the new location of the displaced mass. That, however, is inconsistent with special relativity, which is based on the axiom that all ...
The main takeaways behind Einstein's general theory of relativity: 1. Time and space are neither flat nor fixed; they are curved and distorted by mass and energy. 2. Gravity is not a force, but rather a distortion of time and space. 3.
A controversial new hypothesis that suggests our understanding of gravity is wrong has just passed an important first test. First proposed back in 2010, the new hypothesis states that gravity might behave and arise very differently than Einstein predicted, and an independent study of more than 30,000 galaxies has now found the first evidence to back this up.
Gravity - Experimental Study, Newton, Einstein: The essence of Newton's theory of gravitation is that the force between two bodies is proportional to the product of their masses and the inverse square of their separation and that the force depends on nothing else. With a small modification, the same is true in general relativity. Newton himself tested his assumptions by experiment and ...
gravity, in mechanics, the universal force of attraction acting between all bodies of matter. It is by far the weakest known force in nature and thus plays no role in determining the internal properties of everyday matter. On the other hand, through its long reach and universal action, it controls the trajectories of bodies in the solar system and elsewhere in the universe and the structures ...
Neutron stars. Additional resources. General relativity is physicist Albert Einstein's understanding of how gravity affects the fabric of space-time. The theory, which Einstein published in 1915 ...
Newton Introduces Gravity . The major contribution developed by Sir Isaac Newton was to recognize that this falling motion observed on Earth was the same behavior of motion that the Moon and other objects experience, which holds them in place within relation to each other. (This insight from Newton was built upon the work of Galileo, but also by embracing the heliocentric model and Copernican ...